Authors: Tatjana N. Dujsebayeva and Dmitry V. Malakhov
Abstract: We developed the ecological niche model (ENM) of Ranodon sibiricus in the Dzungarian Alatau Mountains using GIS (Geographic Information System) modeling, and interpretation of orographic and climatic variables. We analyzed the impact of each variable and evaluated the results to discern the most important (key) variables describing the ecological niche. Six orographic and 26 climatic variables were selected as key variables. The analysis of relief and climate contribution to ENM formation reveals a close interrelation between the two factors. The origin of every watercourse, its hydrological parameters, and microhabitat diversity are determined by local microrelief and microclimate. The mean temperature of the warmest quarter, precipitation during the wettest quarter, annual precipitation, and precipitation in the winter months indirectly confirmed the important role of glaciers in maintaining the water balance in streams inhabited by the amphibian. The data acquired allow the new interpretation of the previously proposed idea of introducing the salamander into some regions of Dzungarian Alatau and Northern Tien Shan.
Keywords: Ranodon sibiricus; ecological niche modeling; orography; climate; hydrography; key variables; conservation.
To download full version of article below:
INTRODUCTION:
The number of works dealing with ecological niche modeling (ENM) has notably increased in recent years (Ray et al., 2002; Graham and Hijmans, 2006; Penman et al., 2007; Raxworthy et al., 2007; Suzuki et al., 2007; Tarkhnishvili et al., 2009; Litvinchuk et al., 2010; Doronin, 2015; Groff et al., 2014). This method has proved to be a very efficient way to analyze the geographical distribution of rare species and species characterized by fragmented ranges. ENM utilizes associations between environmental variables and a species’ known locations to abiotic conditions within which populations can be maintained. Obviously, it is a very useful tool for forming both biogeographic conclusions and effective approaches for species conservation.
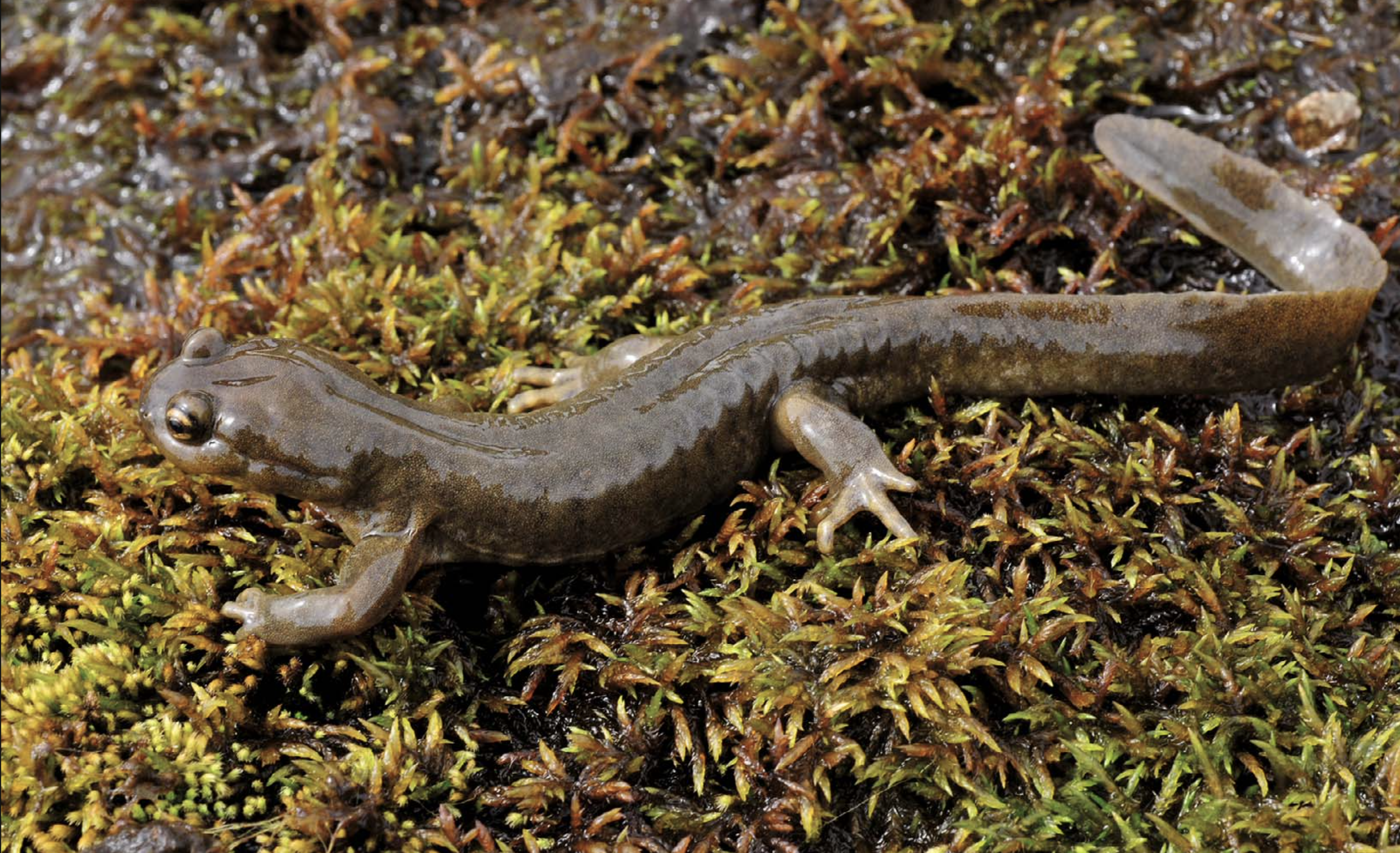
Fig. 1. Semirechensk Salamander (Ranodon sibiricus Kessler, 1866), Keskenterek River Basin (photo by O. Belyalov).
Ranodon sibiricus Kessler, 1866 (Semirechensk Salamander, Xinjiang Salamander) is an endangered urodele amphibian (Fig. 1) that inhabits rivers and brooks in the mountains of the Dzungarian Alatau in southeastern Kazakhstan and northwestern China (Xinjiang) (Kuzmin et al., 2004; Brushko and Dujsebayeva, 2009). There are some indications of the possible presence of the salamander on the Zailiysky (Transili) Alatau Ridge (Northern Tien Shan), both recent (not yet confirmed) and fossil, as Late Pliocene remnants of R. cf. sibiricus have been found (Averianov and Tjutkova, 1995). R. sibiricus is narrowly adapted to many environmental factors and so does not live in every water course of the Dzungarian Alatau (Wang et al., 1992; Kuzmin et al., 1998; Wang and Bai, 2000).
The Semirechensk Salamander was previously known from the northwestern, western, southwestern, and central regions of Dzungarian Alatau (Brushko et al., 1988; Kuzmin et al., 1998; Kuzmin and Tiesmeier, 2001) and later investigations including our careful inspection of 2009 – 2015 added no records outside the previously established range. In China the salamander is known recently from a very limited area of the upper reaches of the Borotala River, where its populations are shrinking both in number and range (Yuan and Zhang, 2006; Chen et al., 2012).
The amphibian inhabits mainly small streams leaking away from glaciers and snowfields and forming a network of first to third-order tributaries of river valleys. Even within the inhabited area the amphibian was found rather accidentally. Its spatial distribution is mosaic in general and sometimes even neighboring streams can differ in abundance of the amphibian.
The problem of the recent spatial and quantitative distribution of the species consists of two parts. The first, obviously, links to the formation of the gross pattern of the species range and the factors that regulated this process in the past. Obviously, the gross pattern of R. sibiricus species range has been developed and honed during the Alpine orogenesis and Pleistocene glaciations (Kuzmin et al., 1998; Malakhov and Dujsebayeva, 2014). However, one may understand past processes only by having a good picture of the current situation, and there is no another possibility (Rautian, 2003). Here, we have tried to solve the second part of the problem by modeling the recent ecological niche of the species and discussing the factors ensuring its wellbeing.
The large scale of the Dzungarian Alatau Mountains, with their complicated and dissected pattern of landscapes, represents the principle obstacle in carrying out a successful ground survey of amphibian habitats. Under such conditions, it is difficult or even impossible to access to every potentially suitable stream by vehicle, horse, or even on foot. Both of these factors — mosaic distribution and inaccessibility — make the GIS approach very useful. Taking into account that the number of factors delimiting the amphibian’s distribution is not very high, and that the animal is not highly mobile, we attempted to develop the model of R. sibiricus‘s spatial distribution using remote sensing data and GIS modeling. We adopted the Hutchinson definition of the ecological niche as an n-dimensional abstract space, with each axis of this space corresponding to a certain parameter (Hutchinson, 1957). The species has a definitive tolerance range in each ecological parameter, in which it could survive (so-called “ecological valence”). The n-dimensional figure, outlined by projections of edge interval points from each factor axis, will represent the ecological niche of a given species. The core of ecological niche modeling is to define the complex of abiotic parameters (temperature, precipitation, relief, etc.) that best describe the potential species distribution in geographic space. This approach allows definition of the most appropriate areas for future species introduction, on the one hand, and revelation of the most vulnerable stages in the species’ life cycle judged from the key factors revealed, on the other. Here we did not take into consideration biotic variables such as inter- and intraspecies relations. That is a more difficult task planned for future research.
MATERIAL AND METHODS
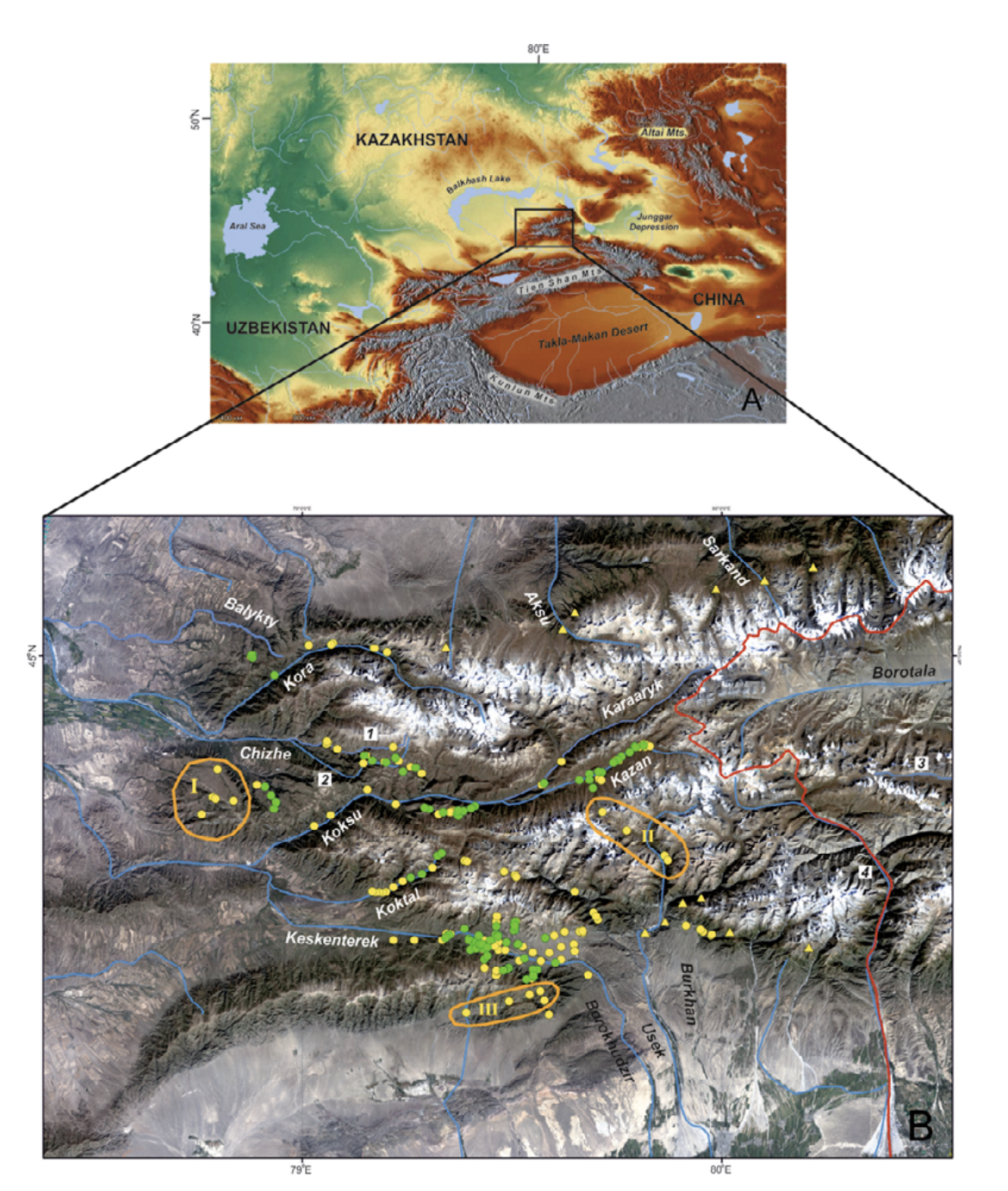
Study area. The Dzungarian Alatau is a mountain system located from 44 to 46°N and from 78 to 82°E in southeastern Kazakhstan and northwestern China, in Xinjiang (Fig. 2). It consists of two main ridges — the Northern Central Ridge and the Southern Central Ridge — and a series of additional less extensive ridges to the north and south. The highest point — Semenov-Tien Shansky Peak, or Besbakan Peak — is 4622 m a.s.l. The glaciers, which have recently been shrinking, are typical for highlands (Vilesov et al., 2013).
The Dzungarian Alatau is surrounded by huge, arid intermontane depressions (the Balkhash-Alakol Depression, Ili Depression, and Dzungarian Basin) to the north and south, is terminated by the Dzungarian Fault in the northeast, and joins the Eastern Tien Shan spurs (ridges) in the southeast (Selivanov, 1965; Didenko-Kislitsina, 2001; Mamedov and Novikov, 2015). The main ridges are divided by the westward-flowing Koksu River and eastward-flowing Borotala River (Fig. 2). The Kongor-Obo Mountains, where the two main ridges converge, are located just by the 80th meridian and the two rivers mentioned start here. Most rivers flowing from the Dzungarian Alatau run into the Balkhash Lake Basin, and the remainder into the Alakol Lake Basin and Borotala River. Most of the rivers are oriented submeridionally, whereas the rivers located within the intermontane depressions have a latitudinal orientation.
Species locality data. We collected the ground-truth points on the amphibian distribution from 2009 to 2014 in the Dzungarian Alatau Mountains where the basins of Balykty, Kora, Chizhe, Chimbulak, Oisaz, Cherkassay, Koktal, Koksu, Keskenterek, Borokhudzir, Maly Usek, Sredny Usek and Burkhan rivers were surveyed at the elevation range from 1300 to 2800 m a.s.l. The water streams, inhabited with amphibian, were the first and second order tributaries of rivers. They were predominantly fed from the glaciers and snowfields placed in their upper flow or supported by underground water (springs) originating from precipitation, and seasonal and glacial meltwaters (Akhmedsafin et al., 1975; Dzakelov and Bazarbayeva, 2015).
In total, we inspected more than 50 water streams and 253 localities within them. We found the amphibians in 111 localities (Fig. 2), which applied in GIS-modeling. Literature data not used in the modeling but discussed in the text we also indicated in Fig. 2. The principle method of field surveillance was day and night hiking routes along the low, mid, and upper sections of every stream and up to several hundred meters long (see Dujsebayeva, 2013 for more details). All the points of Ranodon records were logged with GPS.
Environmental variables and model approach.
Ecological niche modeling is, principally, the procedure of variables weight study to determine the optimal combinations of abiotic factors, delimiting the peculiarities of the animal life cycle. The following variables were taken into consideration: height, slope, aspect, and curvature (derived from Digital Elevation Model), precipitation and air temperature for selected months and quarters (BIOCLIM and WORLDCLIM datasets, http:GGwww. worldclim.org, http:GGwww.worldclim.orgGbioclim), Global Potential Evapo-Transpiration (Global-PET). All variables, represented as rasters with spatial resolution of about 1 km, were analyzed with ArcGIS 10.1.
The method of modeling, applied in this work, could be assigned to as “presence only” It consisted of two major steps: 1) to estimate the range of values within each variable analyzed which best describes the “satisfaction to the requirements of species” and 2) to evaluate the contribution of each variable to actual species distribution. The goal of both procedures was to select the “key” factors that allow developing the map of potential species distribution.
To achieve the goal we randomly divided all ground points collected into the experimental group (75%) and control group (25%). We used the experimental group to define the variable ranges and the control group to discriminate the “key” variables. Both experimental and control ground points were transformed into shape-file and the values of underlying pixels from each variable raster were automatically gathered and registered in the experimental shape-file attributive table. Assuming the normal distribution of variable values by randomly selected points, we cut off the marginal 25% of values (percentiles 12.5 and 87.5) from analysis to left 75% of variable values as an “optimal” range of a given factor.
We reclassified each variable raster in ArcToolbox so that all values, falling within 75% range we converted into value “1,” and values out of 75% range we converted into zero-value. So, new variable raster consisted of cells with only “one” or “zero” value, where “one” means suitable range of a given variable to animal and “zero” was attributed as “non-suitable” range. At this stage, it became possible to summarize all reclassified rasters, or selected sets of rasters, like “precipitation,” to get the general model of potential species distribution. However, the most significant step was to evaluate the importance of each variable. It was obvious that all variables had different impact to animal life cycle. Some factors could appear as triggers regulated the certain stages of life cycle, whereas the other ones did not seriously affect the species biology, or may even were indifferent for animal. It was important to understand, that “suitable” range, defined in previous stage, represented rather probability, expected range of possible species distribution, than the area actually inhabited with animal. The “control” group we used to evaluate the significance of each variable. The shape-file, consisting of control group points, we applied to register “one” or “zero” values of reclassified raster.
The percent of control Point’s General Match (PGM) to the interval “one” was calculated. The median (the number separating higher half of data sample from the lower half) for the entire PGM row was calculated, and those variables demonstrated PGM higher than 73% (median value), were assigned to as “key” factors for the animal (32 of 86 initial variables) and used to develop the potential ecological niche of R. sibiricus.
This model was very basic, as it did not consider many environmental and biotic factors, like the presence of food, water chemistry, or even the presence of streams within suitable area. The potential water flow distribution within the study area could be computed with Spatial Analyst at the base of Digital Elevation Model. However, ArcGIS did not provide the information on actual water presence or absence in potential channels. Further model clarification is possible with attracting of remotely sensed and other sources of spatial information.
Relief classification was based on Svarichevskaya (1965), Zhuchkova and Rakovskaya (2004), Sharaya and Shary (2004), Cherkasov (2004), and Podrezov (2014). There is some controversy in the altitudinal zoning of the mountains of southeastern Kazakhstan as a result of different study specificity (relief, climate, glaciers, etc.). We took 700 – 1300 m a.s.l. (1600 as maximum) as lowland, 1300 (1600) – 2600 (2800) m a.s.l. as midland, and the zone above 2800 m a.s.l. as highland for mountains located in the desert zone (Isachenko, 1985). Among other relief parameters we used a set of curvatures that describe the concavity or convexity of the surface and the consequent tendency of water flow to deceleration-acceleration or divergence-convergence (see explanation for ArcGIS in Blaga, 2012).
Climate analysis mainly relied on the Köppen-Geiger updated climate classification map (Kottel et al., 2006; Peel et al., 2007) with partial use of Vilesov et al. (1986) and Khromov and Petrosyants (2006). The data on the current temperature and precipitation regimes for different altitude zones were borrowed from Podrezov (2014) with corrections for the mountains of southeastern Kazakhstan (Cherednichenko et al., 2013; Dujsebayeva et al., 2014). The toponyms were taken from http:GG sasgis.ruGsasplaneta.
Survey efforts. To check the GIS model, we inspected some additional areas in the Kazakh part of the species range in 2015: (I) the Zhangyz and Kussak rivers (Suyuktobe Mountain — most westerly spurs of the Dzungarian Alatau), (II) the upper and middle flows of the Sredny Usek River, and (III) a small intermontane depression in the northeastern periphery of the Ili Depression (Fig. 2B).
RESULTS
1. Analysis of relief and climate variables
In total, we analyzed 7 relief variables and 79 climate variables and described the modes of temperature, precipitation, and potential evapo-transpiration (PET).
1.1. Relief.
Whenmodelingthereliefoftheecological niche of R. sibiricus we analyzed the altitude, slope, aspect, general curvature, profile curvature, plane curvature, and tangential curvature of the surface. The optimal ranges of variable values are presented in Table 1.
The optimal altitude range of R. sibiricus EN was determined as 1950 – 2400 m a.s.l., which corresponds to the midland of the Dzungarian Alatau. The slope range varied from 3 to 15°. In two-thirds of localities (66.6%) were defined as flattish or weakly sloping surfaces (3 – 9°), 16% as sloping surfaces (9 – 12°), and 17, 4% as strongly sloping surfaces (12 – 15°). The range of aspects was highly wide, excluding the rare slopes with a NNW-NNE aspect (324.3 – 41.6°).
The values of plane curvature corresponded to surfaces ranging from concave (mostly flow accumulation) to weakly convex (low flow divergence). The values of the total profile curvature characterized the surface as flattish or weakly convex. The resulting general curvature was almost zero, corresponding to flat surfaces (Table 1). Accordingly, we described an orographic model of R. sibiricus‘s EN as an almost flat surface located on weakly gentle or gentle midland slopes (1950 – 2400 m a.s.l.) within a wide range of aspects.
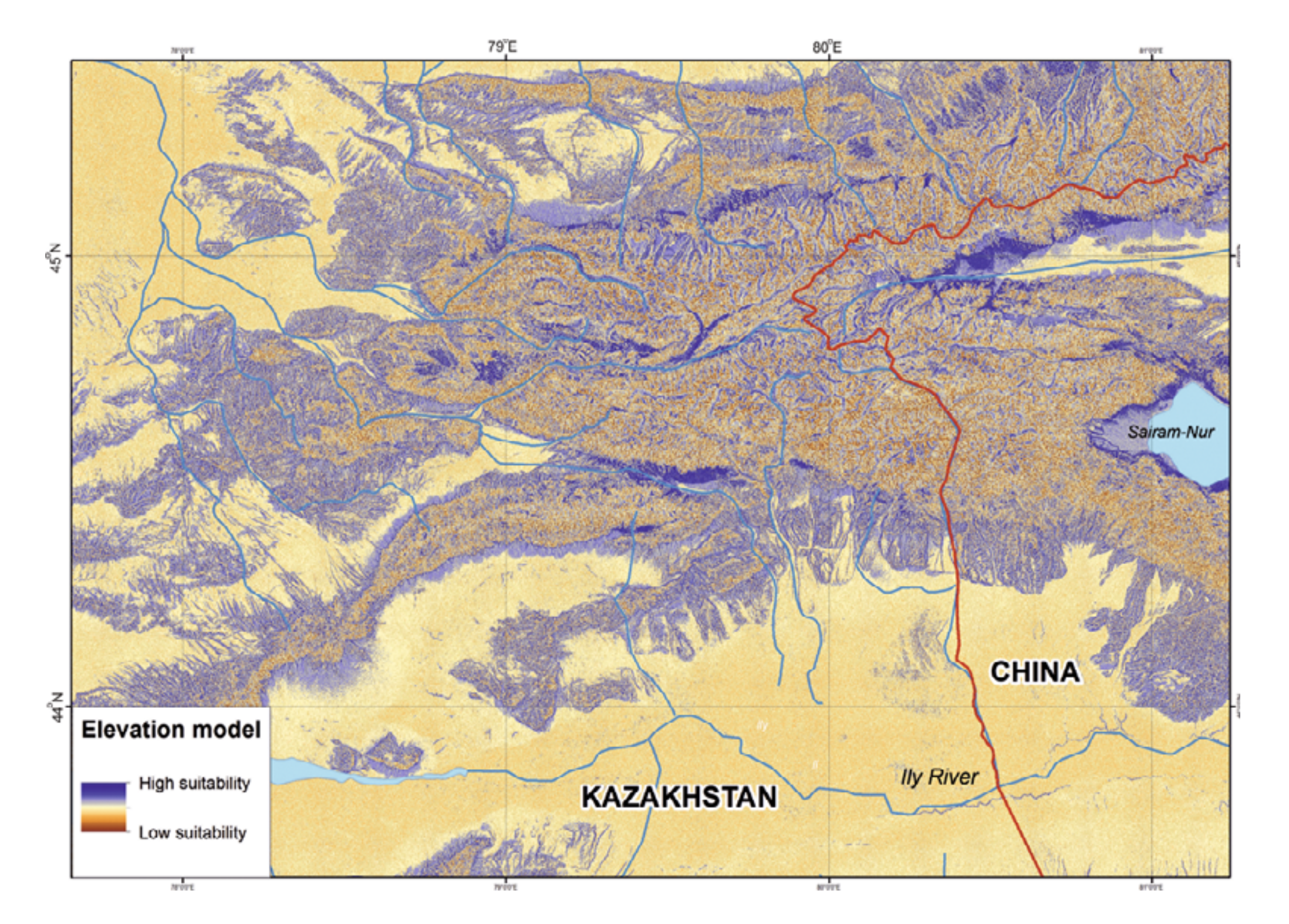
According to our ENM, the following territories in the Kazakh part of the Dzungarian Alatau are most suitable for R. sibiricus: the northern slopes of Minchukur Mountains south of Kapal town (perhaps inhabited by R. sibiricus even in the early 20th century: Shnitnikov [1913]); several sections in the midland of the northern slopes of Northern Central Ridge along the upper reaches of the Bien, Aksu, Sarkand, Maly and Bolshoy Baskan, and Lepsi rivers; the right bank of the middle reaches of the Kora River; the Chizhe River’s sources (the Maly Chizhe and Koitass rivers); the Oisaz and Chimbulak rivers; the upper and (in part) the middle reaches of the Koksu River including its sources (the Karaarik and Kazan rivers) and main tributaries (the Tersakan, Arasan, and Ermensay rivers); the rivers flowing from the Aktobe and Suiktobe mountains (south of Tekeli town); latitudinal sections of the Koktal River in its upper and middle reaches; the upper reaches of the Keskenterek and Borokhudzir rivers; small intermontane depression in the southeast of the Suattau Mountains; and a very limited section of the upper reaches of the Burkhan River (Fig. 3).
In China the most suitable territories could be defined as the Borotala River’s upper reaches, the middle reaches of the Urtaksary River, the northern foothills of the Bedzhintau Ridge, the periphery of the Sairam-Nur Lake Depression, and the headwaters of Kusimchek River (Fig. 3).
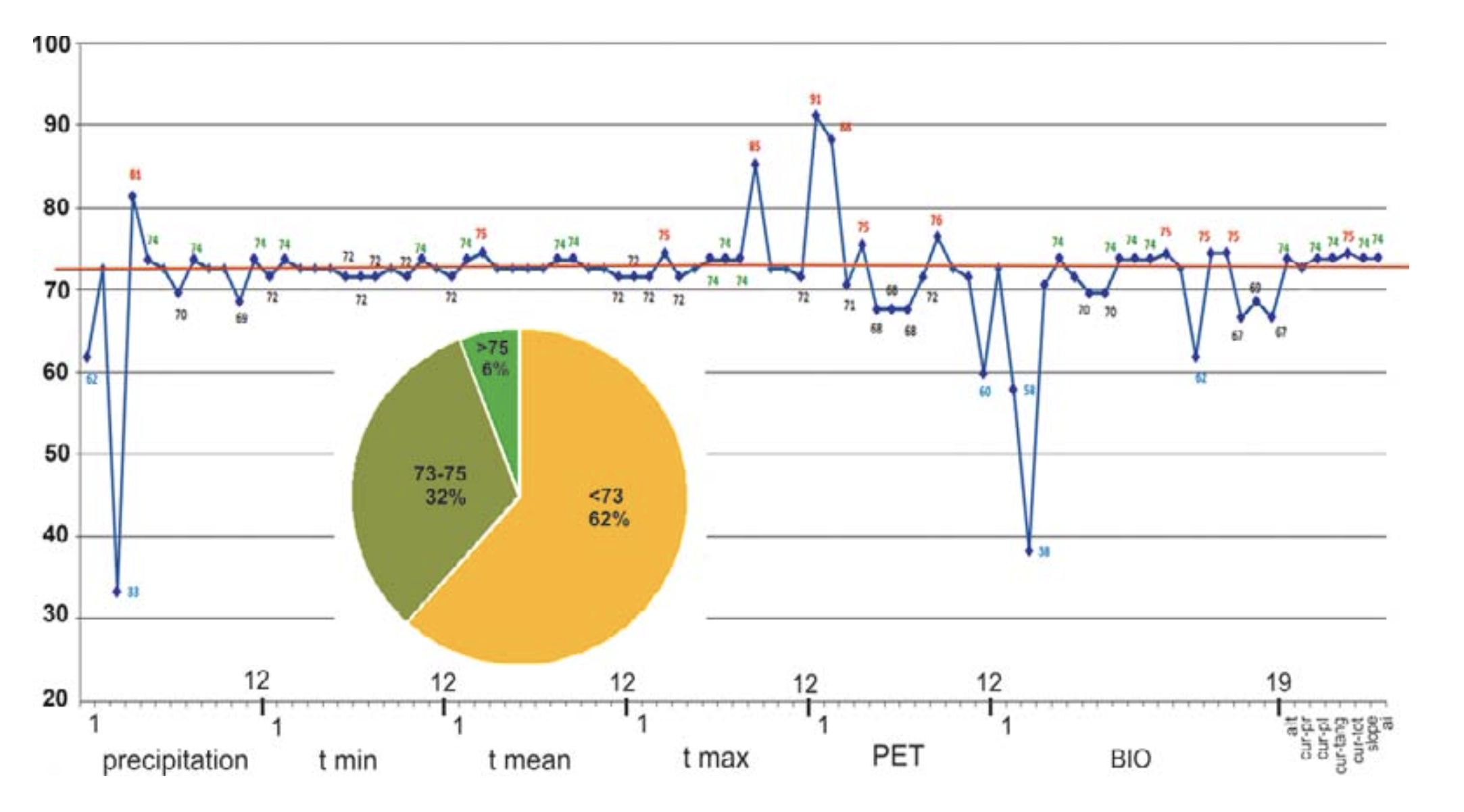
Six of the seven relief (orographic) variables analyzed demonstrate a high coincidence with control group points (Fig. 4). Of these six variables, total curvature has the highest coincidence (75%). Thus, according to our model these variables are of special importance for R. sibiricus EN and are among the key parameters.
1.2. Climate.
According to the model, of the 79 climate variables analyzed 26 could be classed as key parameters, having a significance above the median (>73%). Among these were March mean and maximum temperatures; September maximum temperature; January, February, April, and September PET; annual precipitation; precipitation seasonality; April precipitation; and precipitation in the wettest season (May–July). Of these, four variables — April precipitation, March maximal temperature, and the PETs of January and February — correlated best (81, 85, 91, and 88%, respectively) to the amphibian’s presence (Fig. 4).
Temperature. R. sibiricus EN model was characterized by the following values: a mean monthly temperature range of the warmest quarter (June – August) of +10.3 – 15.9°C), a mean monthly temperature range of the coldest quarter (December – February) from –11.7 to –17.0°C, maximum temperatures in the warmest season of +22.5°C, minimum temperatures in the coldest season of –23.0°C, a mean July temperature of +14.5°C (13.0 – 15.9), a mean January temperature of –16.6°C (15.5 – 17.7), an annual temperature range of 28 – 33°C, and three months with mean temperatures at or above +10°C (Table 2).
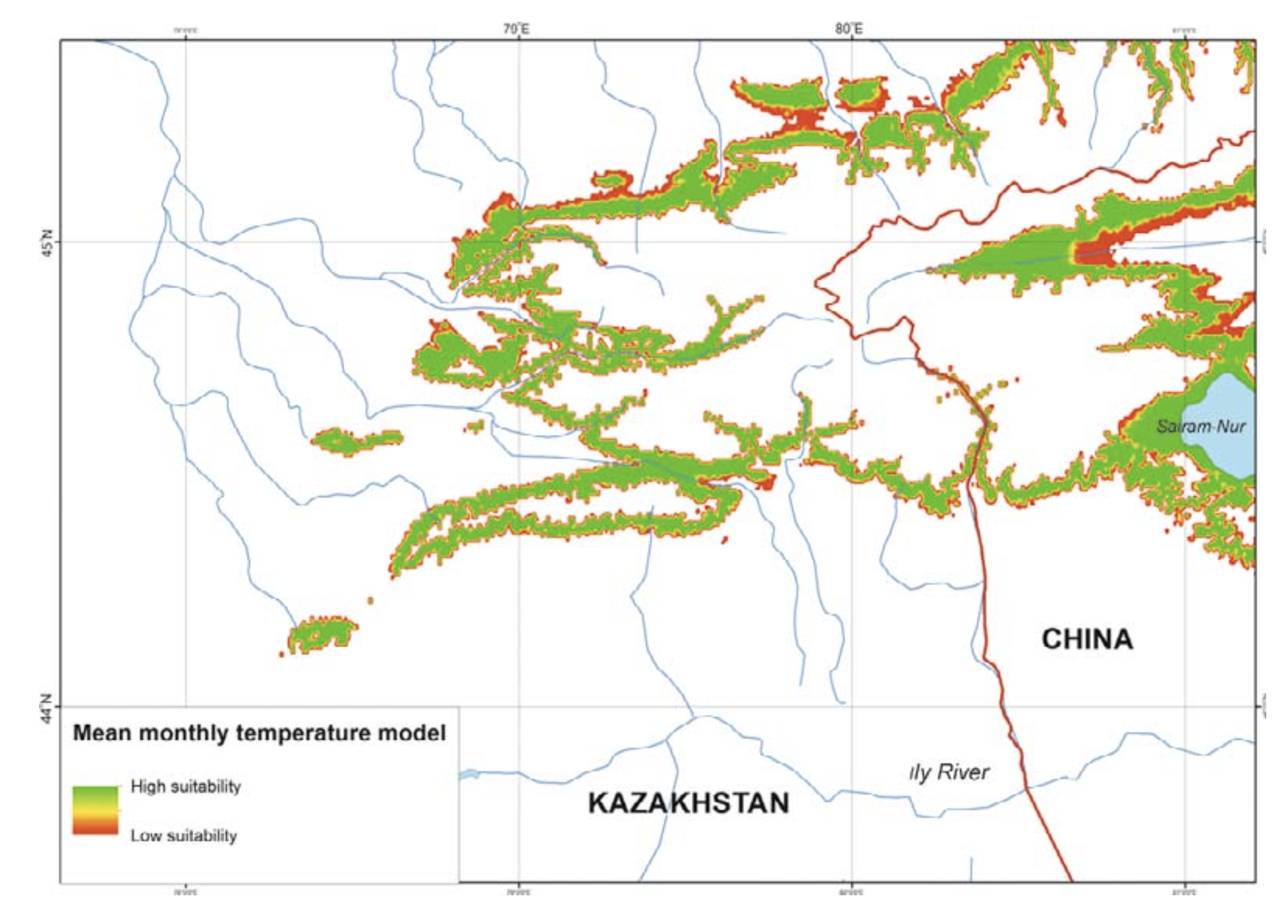
The model’s mean monthly temperatures for the period when the salamander is active (April – September) correspond to the prevailing temperatures of the midland zone in southeastern Kazakhstan (Table 2). The model’s mean temperatures for early spring (March) and mid-autumn (October) corresponded to actual highland conditions. During the cold season (December – February) the model’s mean was lower compared to the prevailing highland conditions and significantly lower (by more than 10°C) than that of the actual midland mean temperatures (Table 2). The modes of maximum, mean, and minimum monthly temperatures had a similar pattern and showed that areas suitable for the species were widely present in the midland of the Dzungarian Alatau (Fig. 5).
According to the high percentage correlation of species records with ranges of optimal temperatures, the March mean and maximum temperatures (75%) and September maximum temperature (85%) from the WorldClim dataset were among the key parameters (Fig. 4). In the BioClim dataset, the key parameters included the maximum temperature of the warmest month (July, 74%) and the mean temperatures of the driest and coldest (December – February, 74%) and warmest (June – August, 74%) quarters.
Precipitation. The ENM was characterized by rainfall throughout the year with annual precipitation of 459 – 504 mm, maximum rainfall in May – July (wettest quarter: 182 – 213 mm) and minimum precipitation in December – February (driest quarter: 55 – 62 mm) (Table 3). From the percentage of species record correlations with optimal precipitation ranges, the April (81%), May, August, and December (74%) precipitation levels from the WorldClim dataset and annual precipitation, precipitation seasonality, and precipitation in the wettest quarter (75%) from the BioClim dataset were classed as key parameters (Fig. 4).
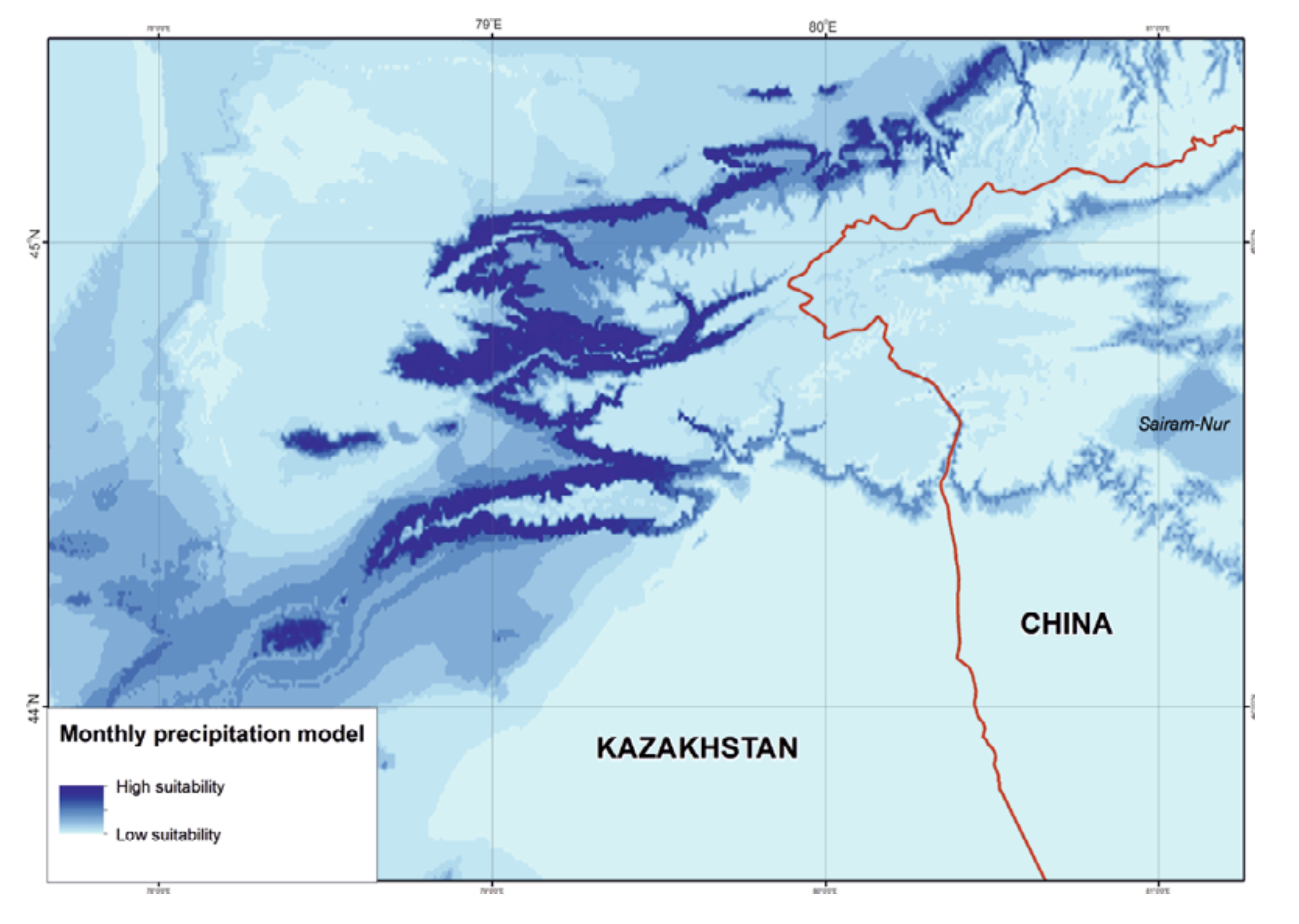
Compared to temperature, the precipitation mode visibly limited the area suitable for R. sibiricus. The western, wettest part of the Dzungarian Alatau was most favorable, unlike the northeastern, eastern, and southeastern regions with minimal rainfall (Fig. 6). However, according to some observations (Kabak, personal communication), several areas within these regions, for example, the wide valleys of the Zheldiaryk and Kumbelsay rivers (the upper reaches of the Khorgos River) with wet meadows and Picea forests seem to be quite suitable for the salamander.
Potential evapo-transpiration. The ENM was characterized by the predominance of PET over precipitation both in total (646 – 736 mm against 459 – 504 mm) and through April – October. The PET maximum was in July (129 – 142 mm) (Table 3). PET and precipitation equilibrium occurred in October and PET minimum in January-February (0 – 4 mm). The PET for January and February (91 and 88% of matches, respectively), April, and September (75 and 76%, respectively) were classed as key parameters (Fig. 4).
1.3. Ecological niche model.
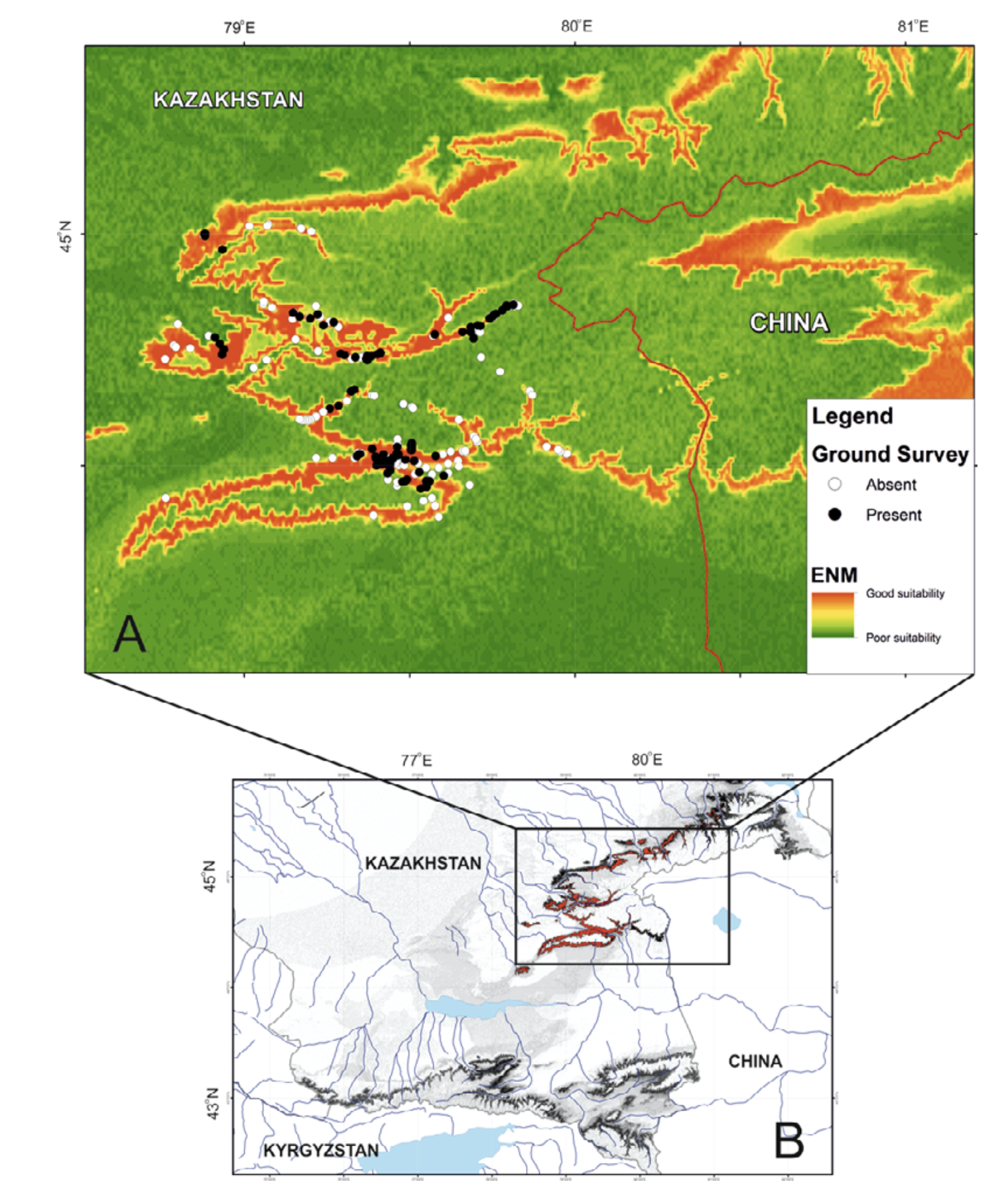
We have built the resulting R. sibiricus ENM with key variables. As shown in Fig. 7, the best conditions for the salamander in the Kazakh part of the Dzungarian Alatau exist in the following regions: the upper reaches of the Borokhudzir and Keskenterek rivers, the middle reaches of the Koktal River, the upper and part of the middle reaches of the Koksu River (including the Karaaryk and Kazan sources), and in Chizhe River including the Chimbulak and Oisaz sources (Fig. 7A). In China, the most suitable area is in the upper reaches of the Borotala River in a longitudinal range between the tributary Chonbulak and Aksai rivers in the west and the Tzagan-Us and Big Bezgin rivers in the east (80°15¢ – 80°30¢ E).
In Kazakhstan lower habitat suitability was found in the midland of the Northern Central Ridge in its western and central parts, the upper and middle flows of the Usek and Kora river basins, some areas in the Koktal — Koksu and Chizhe — Kora river watersheds, the Minchukur Mountains, the extreme western spurs of the Dzungarian Alatau, and the Altyn-Emel Ridge in the south (Fig. 7A). The last is no longer inhabited by R. sibiricus. However, there is information on the species being found in the Bizhe River’s sources flowing from the northern slopes of the Altyn-Emel Ridge in 1941 (Paraskiv, 1953). In China, the upper reaches of the Borotala River within the area of 80°30¢ – 80°50¢ E, the Urtaksary River in part, the western and southern coasts of Sairam-Nur Lake, and the Kusimchek, Dzhergalan, and Ashaly (Achal) rivers showed lower habitat suitability for R. sibiricus (Fig. 7A).
The lowest habitat suitability was seen in the midland of the northern (Kazakhstan) and southern (China) slopes of the easternmost Northern Central Ridge, the midland of the Burkhan, Tishkan, Chizhin, Khorgos, Kigen’, and other rivers flowing from the Toksanbay (Kazakhstan) and Bedzhintau (China) mountains and small spots within the extreme western Boro-Khoro Ridge (Fig. 7A). The Zailiysky Alatau Ridge, Kungey Alatau, Ketmen Ridge (all part of the Northern Tien Shan), and Central Tien Shan mountains — along with Narat Ridge — also came into the “low suitability conditions” category (Fig. 7B).
DISCUSSION
Of the abiotic factors applied to analysis of amphibian ENs, more attention has always been paid to relief and climate. For EN modeling in mountain areas, altitude and (less often) slope were more widely used from among the orographic variables and precipitation from the climatic ones (Suzuki et al., 2007; Tarkhnishvili et al., 2008, 2009; Litvinchuk et al., 2011). More than once, researchers have tried to reveal the abiotic factors defining the distribution pattern of R. sibiricus. Bannikov (1949) connected the occurrence of the highest density of salamanders in the midland of the Dzungarian Alatau with maximal precipitation at 2000 m a.s.l. Kuzmin et al. (1998) defined slopes of from 80 to 160 mGkm as corresponding to the flattest areas, and most preferred by the species. These authors confirmed the importance of maximal precipitation in the midland of the Dzungarian Alatau and emphasized a close relationship between precipitation, temperature regime, and vegetation. Kuzmin et al. (1998) described secondand third-level river tributaries flowing over flattened areas in the coniferous forest zone at 1450 – 2650 m a.s.l. as “typical” R. sibiricus habitat. Borkin and Litvinchuk (2011) applied the Maxent Program to designate a hypothetical range for Hynobius turkestanicus in the Alay Region (Central Asia). They used GPS-recorded localities of two hynobiid species Paradactylodon mustersi (6) and R. sibiricus (28), data on their altitudinal distribution and 19 BioClim variables (BIO 1 – 19) from the WorldClim dataset. They established the altitude, precipitation in the driest month, and mean annual precipitation as the main factors affecting the species distribution.
We analyzed 7 orographic and 79 climatic variables, revealed the key variables (Fig. 4), defined their optimal ranges, and built the model of R. sibiricus EN (Fig. 7).
Relief. R. sibiricus ENM was described as flat or flattish surfaces located on gentle slopes of midland at 1950 – 2400 m a.s.l. Such a pattern coincident with the Kuzmin et al. (1998) intimation on flattened mountain plateaus as a habitat preferable by this species. Figure 3 shows that this description defines intermontane depressions with rivers of sublatitudinal stretch, or parallel orientation to the main axis of the conjugate ranges (Edelshtein, 1947; Kostenko, 1975). In the Dzungarian Alatau, the basins of the Chizhe, Koksu (in the middle and upper reaches), Borotala, Keskenterek, and Borokhudzir (in their upper reaches) rivers are situated in that type of depression (Fig. 2). The basins of these rivers possess the highest R. sibiricus populations (Brushko et al., 1988; Kuzmin et al., 1998; Dujsebayeva, 2013).
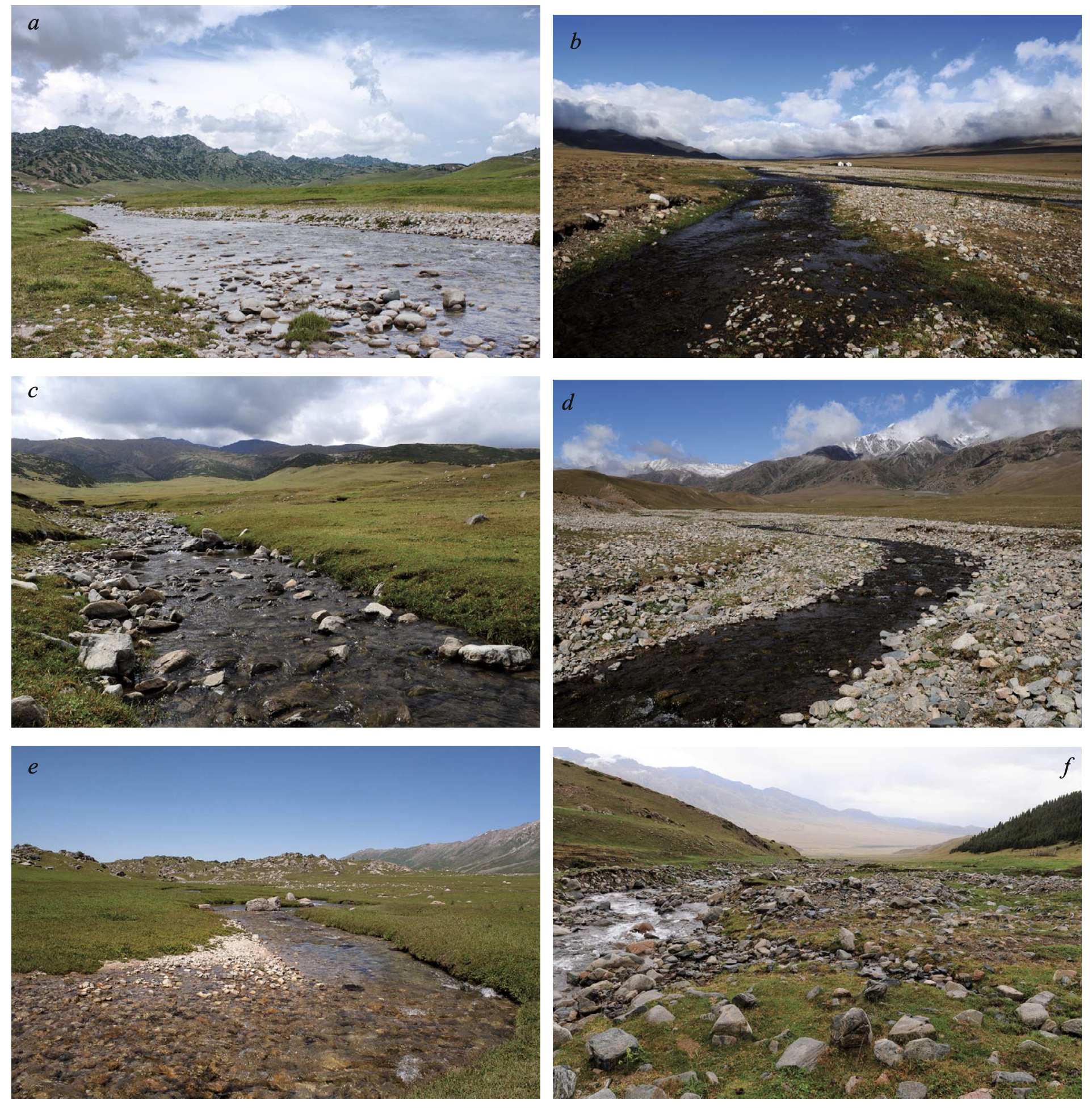
The rivers of sublatitudinal stretch are distinguished by widely expressed floodplains, absence of erosive slopes, a straight or slightly sinuous mainstream with gentle lengthwise riverbed, and relatively even width without flexures and rifts (Fig. 8a – e). The river network in general is very complex and well branched. Numerous tributaries reach the mainstream across gentle slopes (Kalesnik, 1932; Edelshtein, 1947; Borisevich, 1958; Zhandayev, 1972, 1984). Low surface curvature and slope flatness are expressed in not high speed of water streams. Usually, the substrate of the bottom and banks is well rounded and the shore overgrown with turf.
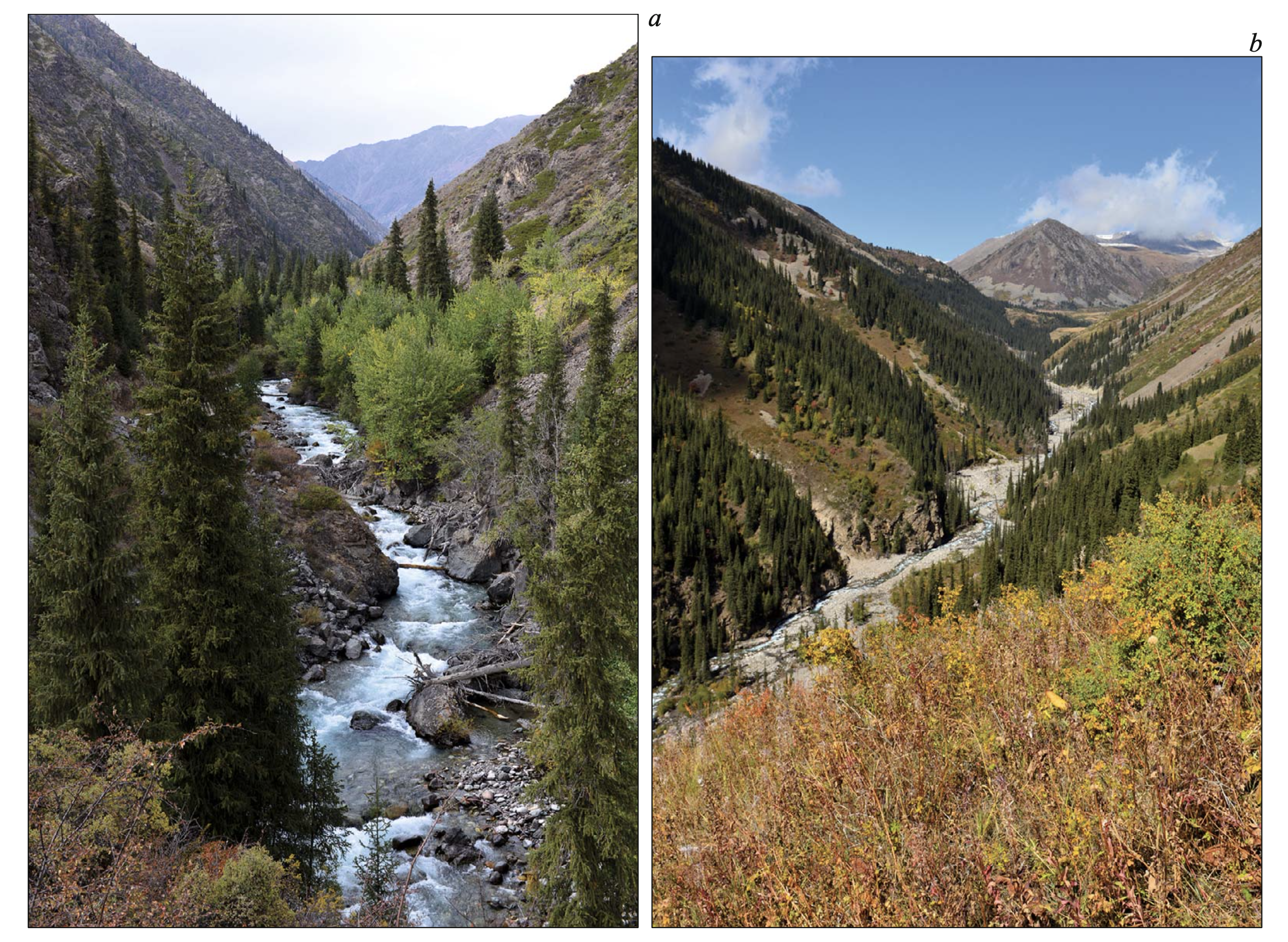
Another type of stream is presented by rivers flowing in gaps or canyons of sublongitudinal stretch or oriented transversally to the main axis of the conjugate ranges (Edelshtein, 1947; Kostenko, 1975; Leontyev and Rychagov, 1988). Sublongitudinal rivers are characterized with absence of any floodplain (or its weak development as narrow strips of pebbles and boulders). They have highly erosive slopes partially indicated by roughly rounded pebbles and boulders of river bottom and banks. Numerous flexures, rifts, rapids, and uneven width of the watercourse are typical for the canyon-like rivers (Fig. 9). The river network is weakly branched. The stair-step mouths of few tributaries reach the mainstream by strongly sloping or even steep surface (Kalesnik, 1932; Edelshtein, 1947; Borisevich, 1958; Zhandayev, 1972, 1984; Podrezov, 2014). In such the rivers R. sibiricus is absent (Fig. 2). The information on salamander finding in the Khorgos River is doubtful, as was noted earlier by Kuzmin et al. (1998).
Orographic ENM shows that certain areas within canyon-like rivers possess features quite suitable for R. sibiricus (Fig. 3). The previous researches provided a special inspection of Aksu, Sarkand, Tentek, Maly, Sredny, and Bolshoy Usek rivers in nineties of 20th century (Kuzmin et al., 1998) to check expanded and flattish spot areas within their beds. We have repeatedly examined the Maly and Sredny Usek rivers from their sources (near 3000 m a.s.l.) to the lowland zone (1300 – 1600 m a.s.l.) but the salamander was never found there (Fig. 2).
Actually, the presence of expanded and flattish small areas within the canyon-like river valleys is connected with the presence of geologically weak zones (increased fracturing of rocks and their intense fragmentation) but doesn’t determine the geomorphological structure in general (Zhandayev, 1960; Kostenko, 1970). Such very limited areas are intensely developed by different erosive factors and resemble the intermontane valleys only convergently.
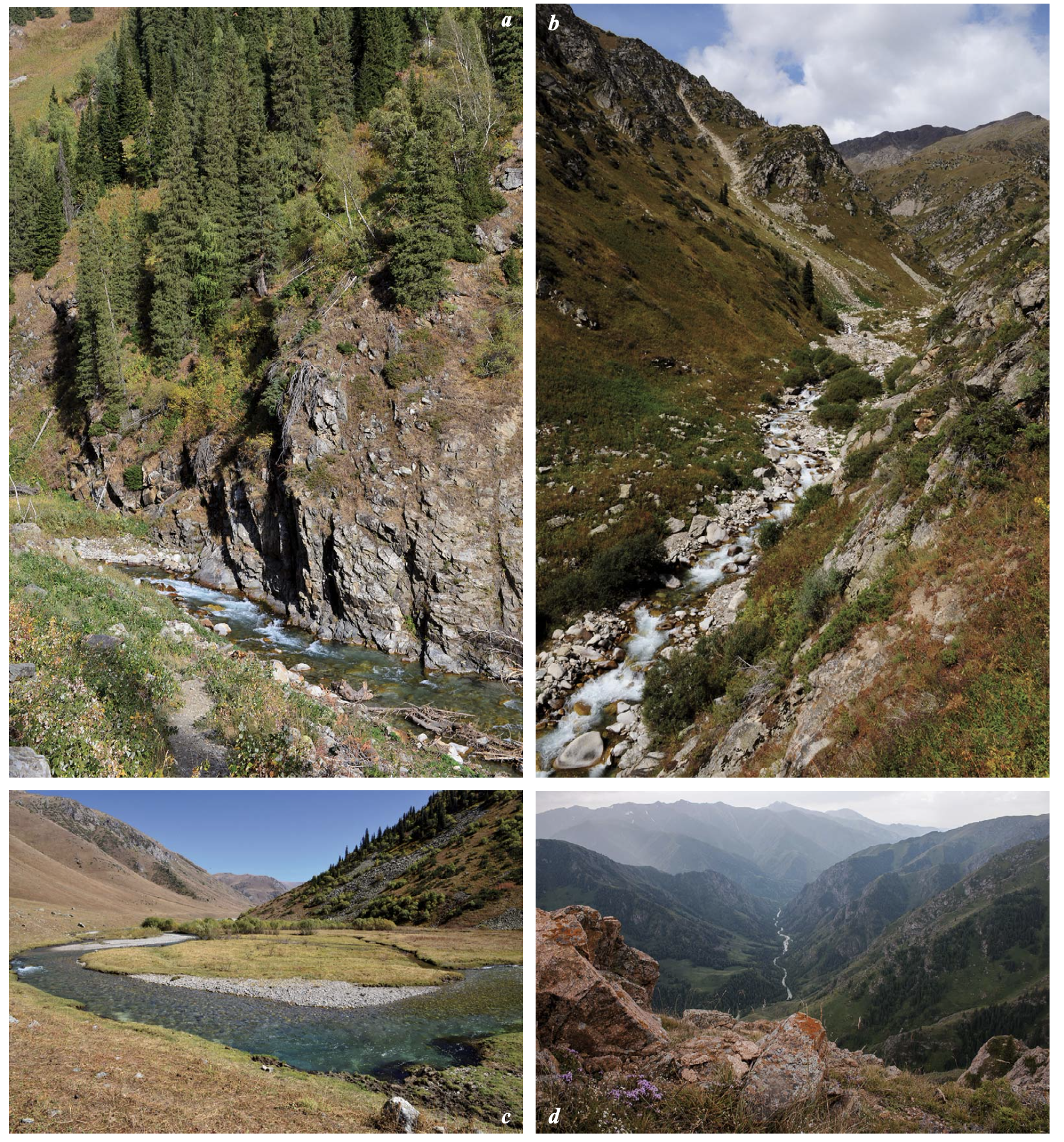
The Kora and Koktal rivers are placed in diagonally stretching valleys, or oriented obliquely to the main axis of the conjugate ranges (Edelshtein, 1947). For such rivers, the characters of transversal or canyon-like streams are more typical but certain sections demonstrate some features of longitudinal valleys (Fig. 10). Not numerous populations of R. sibiricus sporadically inhabit such the rivers (Fig. 2).
Climate. The ENM was characterized by a mean temperature for the warmest month of +13.0 – 15.9°C, a mean temperature for the coldest month of from –15.5 to –17.7°C, a short summer (three months with mean temperatures at or above 10°C), precipitation throughout the year with an annual total of 459 – 504 mm and highest amounts in summer, and an annual temperature range of 28 – 33°C, corresponding to an aridity index of 30 – 50% (Vilesov et al., 1986).
According to that description, the climatic ENM could accord with either the Temperate Continental Climate of Khromov and Petrosyants (2006) or the Humid Continental Climate with short mild-cool summer, relatively cold snowy winter, and all-year precipitation from Köppen’s climate classification (DfbGDfc: Kottel et al., 2006; Pidwirny, 2011). This climate promotes the development of forest vegetation. The streams inhabited by R. sibiricus are located mainly in coniferous forests with endemic Picea schrenkiana and forest-meadow and forest-meadow-steppe belts mainly with Juniperus pseudo-sabina (Goloskokov, 1984).
Concerning the temperature modes (minimal, mean, and maximum), the model does not reveal any significant differences between the river basins studied. In particular, there were no visible differences in temperature variables between the rivers of sublatitudinal and sublongitudinal orientation. In most cases, both groups had similar temperature ranges in the midlands of the Kazakh and Chinese parts of the Dzungarian Alatau (Fig. 5).
In contrast, precipitation is very uneven within the Dzungarian Alatau with significantly higher amounts in the western part of the mountain system and a gradual decrease towards the east. The predominance of moist air masses of northwestern, western, and southwestern origin determines the maximal precipitation in the western part of the Dzungarian Alatau. Along the wide and open intermontane depressions where the Chizhe, Koksu, Koktal, Keskenterek, and Borokhudzir rivers lie, moist air flows easily and can penetrate deep into the interior of the Dzungarian Alatau, where the territories with the main R. sibiricus populations are found (Brushko et al., 1988; Kuzmin and Tiesmeier, 2001; Dujsebayeva, 2013).
The southern (Xinjiang) slope of the Northern Central Ridge, the Bedzhintau Mountains, the Borokhoro Ridge, and some surrounding areas are distinguished by minimal precipitation (Fig. 7). The screening effect of the elevated Northern and Southern Central Ridges with their major centers of glaciation, on one side, and the close proximity of the vast arid Dzungarian Basin, on the other, determine the highly continental and arid climate and weak development of any river network (Didenko-Kislitsina, 1965, 2001; Selivanov, 1965; Sosedov et al., 1984; Cherkasov, 2004; Vilesov et al., 2013). According to Köppen’s climate classification (Kottek et al., 2006; Peel et al., 2007), most of these regions belongs to the semi-arid steppe climate zone (Bsk). The salamanders live in only small numbers in several localities along the Borotala River (Wang et al., 1992; Kuzmin and Tiesmeier, 2001; Yuan and Zhang, 2006; Chen et al., 2012).
With regard to both annual precipitation and its distribution during the species’ period of activity (April – October), the ENM lies between the actual foothill and midland precipitation modes typical for mountains of southeastern Kazakhstan with annual precipitation closer to the foothill mode than the midland one (Table 3). The model also showed the severity of the winters. This was expressed in very air low temperatures and low precipitation (Tables 2 and 3) that were more typical of the climate of arid highlands or arid deserts (Khromov and Petrosyants, 2006). However, we suspect that R. sibiricus shelters in spots that remain unfrozen: bank niches covered by soil, snow, dense moss, and hummocky vegetation (Paraskiv, 1953; Kuzmin, 2012; Dujsebayeva, unpublished data).
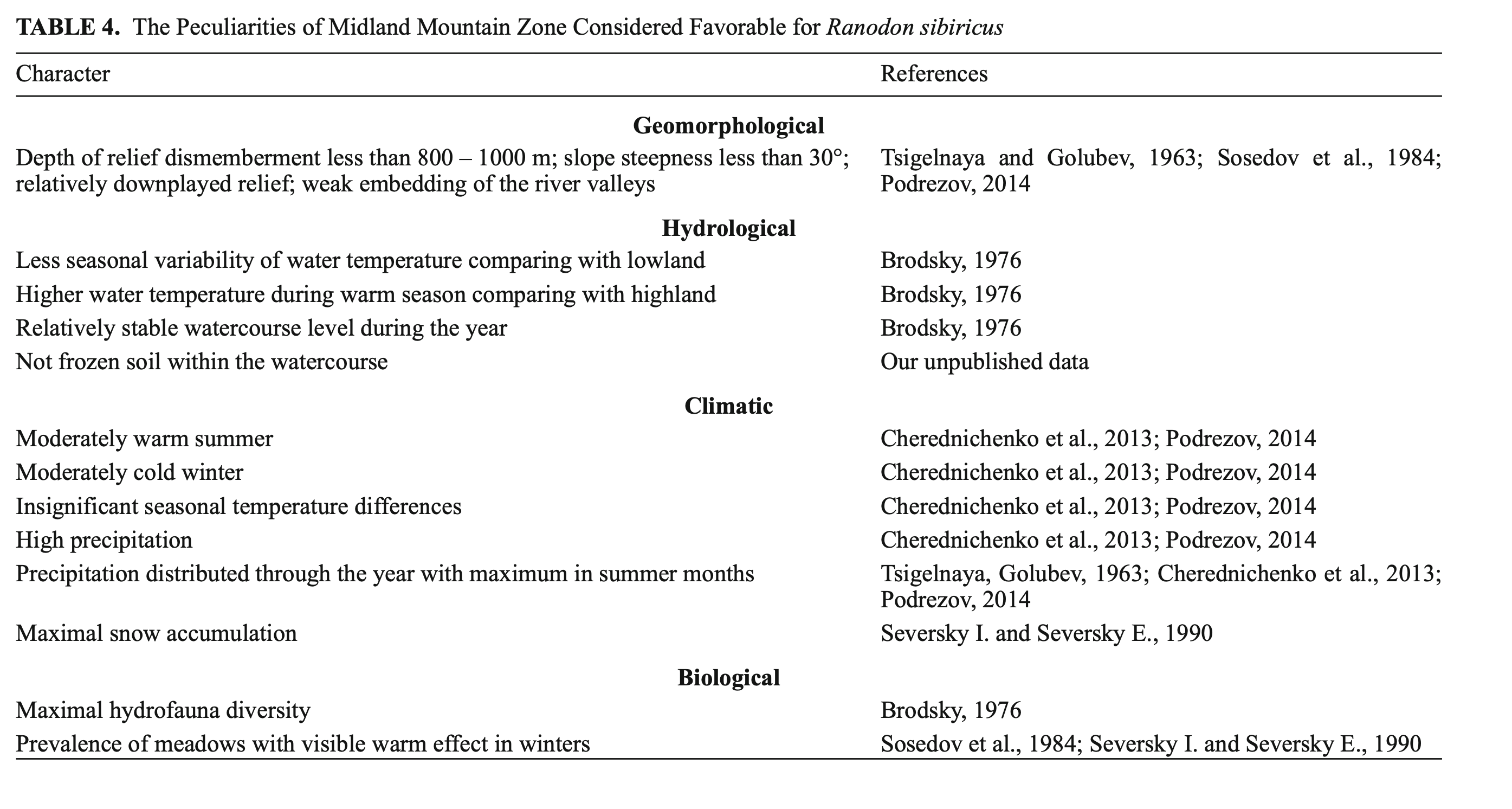
As seen from Table 4, the midland conditions are most suitable for R. sibiricus habitats according to many of the orographic and climatic variables. The maximum altitude for R. sibiricus habitats in Kazakhstan is 2500 – 2600 m a.s.l. with lower results for the northern slopes. The salamander lives at much greater elevations in Xinjiang (up to 3200 m a.s.l.: Wang et al., 1992; Kuzmin et al., 2004; Chen et al., 2012). This follows from the geomorphological peculiarities of the region including the significant influence of the hot and dry lowland of the Borotala River Valley (Murzayev, 1966; Peel et al., 2006). The recent lowest known elevations for the salamander are at 1560 m a.s.l. (our data) in Kazakhstan, and 2000 – 2100 m a.s.l. in China (Kuzmin et al., 2004; Chen et al., 2012).
The higher altitudes are not suitable for normal salamander activity, primarily in the temperature mode. At high altitudes, the water temperature is low even after midday at midsummer. Our data showed that at midsummer daily water temperature usually did not rise above +7 – 10°C at 2500 m a.s.l. and fell to 0.5 – 1°C at night. Unstable weather is typical for highland during most of the year. Sudden and sharp changes in weather are not rare even in summer. We repeatedly registered sharp falls in air temperature (down to +5°C and below) accompanied by snow and hail at midday in June at 2600 – 2800 m a.s.l. in the Borokhudzir River Valley located in the southern periphery of the species range. In the Koksu River Valley (2500 m a.s.l.), the night air temperatures during the warmest month (July) frequently dropped below zero before sunrise. Persistently low temperatures in the highland and significant soil freezing are additionally promoted by frequent and strong winds, large coarse soils and the scarcity of plants (Seversky I. and Seversky E., 1990; Gorbunov et al., 1996).
Obviously, all of these climatic characteristics of the highland curtail the salamander’s daily and seasonal activity and inhibit normal amphibian ontogenesis. The latest egg clutches we observed were in the brooks of the Borokhudzir River at 2446 – 2560 m a.s.l. in late August – early September of 2011. At lower altitudes (2080 – 2115 m) in the same brooks, the egg clutches were already empty and newly hatched larvae were swimming in the water by this time.
The lowland and foothills of the Dzungarian Alatau adjoining large arid depressions and plains are also unsuitable for the salamander (Fig. 2A). These zones are characterized by very high summer air and water temperatures, large daily temperature differences, large annual temperature range and poor precipitation (Tables 2 and 3). Such conditions affect R. sibiricus physiology. The species can generally maintain meaningful activity at a rather low temperature (Kuzmin, 2012). However, it is a typically moisture-sensitive amphibian, requiring water to survive, and the most important environmental variable upon which its mode of life centers is the availability of water (Heatwole, 1983). High air and water temperatures and insufficient precipitation cause significant fluctuations in watercourse levels, even their complete drying during hot andGor waterless years, rendering the habitat unsuitable for the species.
Oroclimatic and Orohygrographic Factors of Ranodon sibiricus Ecological Niche
Climate and relief are interlinked, especially in mountain areas. “Mountains … give rise to a wide range of meteorological phenomena and distinctive climatic characteristics of consequence for ecology, forestry, glaciology and hydrology” (Barry, 2008: 3). The mosaic character of relief in mountains creates a microclimatic diversity that is incomparably more prominent than in valleys (Podrezov, 2014). The hydrothermal regime, encompassing temperature variability, precipitation, the relationship between moisture and evaporation, the balance of solar radiation, etc. of a particular mountain area depends directly upon its relief peculiarities, i.e. elevation, slope and exposure, surface curvature, and so on.
The analysis of the relationship between relief and climatic factors is difficult enough with regard to terrestrial mountain inhabitants but it appears to be even more so when we examine the inhabitants of mountain streams. The relief determines the peculiarities of the hydrographic network in general and every river or brook in particular. The microbiotopic features of a given watercourse depend upon the local microrelief and microclimate. Thus, it would be more correct to talk about “oroclimatic” and “orohydrographic” factors (Gerasimov, 1959; Kostenko, 1975; Podrezov, 2014).
Assuming a close interaction of all abiotic factors, our model confirms the leading role of relief-forming factors in the development of a gross pattern of spatial and quantitative species distribution (Malakhov and Dujsebayeva, 2014). Among climatic factors, precipitation appears most important for R. sibiricus, but it is the relief that largely regulates the pattern of precipitation in mountain areas.
Relief. The median distribution revealed a high degree of correlation of control points (>73%) with all relief parameters (Fig. 4). These parameters were assumed as the “key parameters” and it was supposed that changes in “key parameters” would either directly affect the species’ life conditions (habitat suitability) or be manifested through changes in hydrological and climatic conditions. Relief-forming parameters were closely related to each other.
The slope and curvature regulate runoff capacity (its volume and velocity) (Sharaya and Shary, 2004; Shary, 2006). They define the suitability of a watercourse for amphibian life. According to our data, the speed of watercourses flowing over flat or flattish surfaces within gentle midland slopes corresponds to 10 – 70 cmGsec. The width of the watercourse is not as important and varies significantly. R. sibiricus successfully inhabits small brooks (20 – 50 cm in width) as well as wide rivers (5 – 7 m and more: Fig. 8). It does not live in rapid and powerfully flowing rivers. The rare sightings reported of the salamander in such waters are the result of its being washed downstream (Kuzmin et al., 1998; Dujsebayeva, 2013).
The slope, curvature, and aspect determine the insolation and ensuing surface heating or cooling (Barry, 2008; Podrezov, 2014). Together with precipitation, these factors regulate the watercourse level and snow accumulation and predetermine the extent and duration of snow cover during the cold months thereby affecting the soil’s freezing. They also determine the nature of spring floods and glacier runoff (Seversky I. and Seversky E., 1990; Barry, 2008; Podrezov, 2014). These processes are essential for successful winter hibernation and awakening in spring for the amphibian.

In general, there is a direct link between altitude and the physiological adaptation of aquatic animals through the regulation of oxygen content, but in mountain watercourses the oxygen saturation is almost 100% (Brodsky, 1976). More important for the model is the fact that through interaction with other orographic and climatic factors altitude determines the microclimatic peculiarities of R. sibiricus habitats including food supply (Table 4). According to the ENM, the altitudinal optimum for R. sibiricus in the Kazakh part of Dzungarian Alatau is 1950 – 2400 m a.s.l. (Table 1). Our data showed that the tributaries of the Borokhudzir and Koksu rivers above 2400 – 2500 m on the northern slopes, and 2600 – 2700 m on the southern ones, were free of hydrofauna.
Climate. Our ENM confirmed the previous view of the importance of the temperature and precipitation modes for the well-being of R. sibiricus (Bannikov, 1949; Kuzmin et al., 1998; Kuzmin and Tiesmeier, 2001; Borkin and Litvinchuk, 2011). Of 79 climatic variables analyzed, 26 (33%) were located above the median (>73% match) (Fig. 4).
As regards temperature variables, we determined the mean and maximum temperature of March, maximum temperature of September, maximum temperature of the warmest month (July) and warmest quarter (June – August), and mean temperatures of the driest and coldest quarter (December – February) as the key parameters (Fig. 4).
In the Tien Shan, snowmelt begins in March and the air temperature determines the rate of snowmelt, the power of the spring floods, and the resulting degree of riverbed erosion (Tsigelnaya and Golubev, 1963). A significant rapid rise in the air temperature in March stimulates the exit of salamanders from hibernation but also leads to forceful spring floods that might be dangerous for active amphibians, both adults and larvae (Brushko and Narbayeva, 1988). Seemingly, low air temperatures during this period can only slow down the spring processes. It is known that highest degree of soil freezing is observed before snowmelt (Tsigelnaya and Golubev, 1963), i.e. before the salamanders wake up. Our data on the all-year dynamics of water temperatures in sites of potential R. sibiricus hibernation have shown minimal but still positive water temperatures of +0.5 – 1°C in February – March. This means that watercourses inhabited by R. sibiricus do not freeze and this salamander hibernates in “soft” conditions compared, for example, with Salamandrella keyserlingii (Berman, 2002). However, the influence on the salamander of low March air temperatures coupled with poor snow accumulation has not yet been evaluated.
The warmest quarter of year (June – August) is the primary period of egg-laying and for the development of the clutch and newly hatching larvae, but lower air and water temperatures during this time does not visibly affect these processes. Adults, larvae, and eggs are very resistant to low temperatures and even to brief freezing (Paraskiv, 1953; Brushko, personal communication). Increases in temperature (especially of water) are more risky for the salamander. Brushko and Narbayeva (1988) reported a water temperature above 22°C to be lethal, at least for the larvae of R. sibiricus. We found neither adults nor larvae in streams with a water temperature above +24°C.
Long-term increases in summer air temperatures, together with a deficiency in precipitation, noticeably promote glacier degradation and a reduction in runoff in glacial rivers. This has been observed in recent decades in the Northern Tien Shan and Dzungarian Alatau (Vilesov and Uvarov, 2001; Vilesov and Morozova, 2008). Although no serious problem is foreseen with decreases in the runoff of large mountain rivers in Central Asia in the near future because of the presence of compensatory mechanisms (Severskiy et al., 2006), the situation with small river tributaries remains unevaluated.
The Model of Ranodon sibiricus Ecological Niche: GIS and Remote Sensing Approach 185
The same tendencies in temperature and precipitation are of concern with regard to springs originating in underground mountain waters, the balance of which depends on precipitation, meltwaters (both seasonal and glacial), and, to a lesser degree, runoff. Our sporadic observations in the Borokhudzir River Valley showed a halving in the width and depth of small springs under increased air and water temperatures coupled with poor winter and spring precipitation in 2013 compared with 2010 and 2011. This process was more pronounced on the southern slopes where many springs had completely dried up by the end of summer.
The coldest and the driest quarters in the Dzungarian Alatau coincide over the winter months (Tables 2 and 3). The accumulation and preservation of snow cover during this quarter is important for R. sibiricus‘s hibernation. Sufficient and stable snow cover mitigates the low winter temperatures and prevents deep and long soil freezing (Osokin and Sosnovsky, 2015). Moreover, seasonal snow contributes to the formation of the seasonal glacial snow store, which makes up most of the summer glacier runoff in the Northern Tien Shan and Dzungarian Alatau (Vilesov and Uvarov, 2001; Severskiy et al., 2006).
Precipitation. In comparison to temperature, noticeably more precipitation parameters were found among the key ones, based on percentage matches. They were annual precipitation, precipitation in the spring and summer months from April to August, precipitation in December, and the seasonality of precipitation (Fig. 4, Table 2).
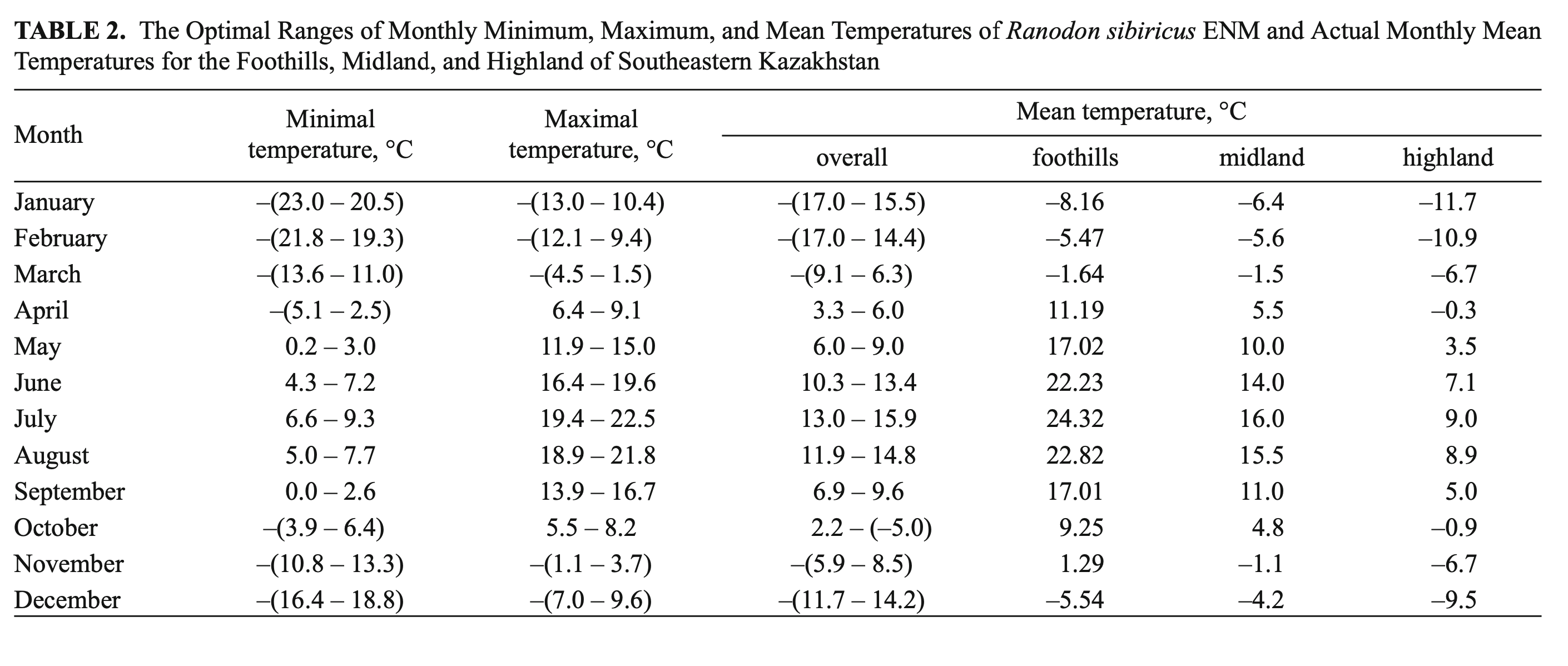
Precipitation from April to August regulates watercourse levels between the end of the seasonal snowmelt (March – April) and the beginning of active seasonal glacier melting (July – August). It also contributes significantly to the conservation of the seasonal glacial snow store that begins actively melting in late summer (Tsigelnaya and Golubev, 1963; Severskiy et al., 2006). Seasonal winter precipitation contributes to glacial store conservation. It constitutes up to 70% of river runoff in the high mountains of the Northern Tien Shan and Dzungarian Alatau (Sosedov et al., 1982; Seversky et al.,
2006). Precipitation in the initial winter period (November – December) contributes to seasonal snow cover and regulates soil freezing (Osokin and Sosnovsky, 2015).
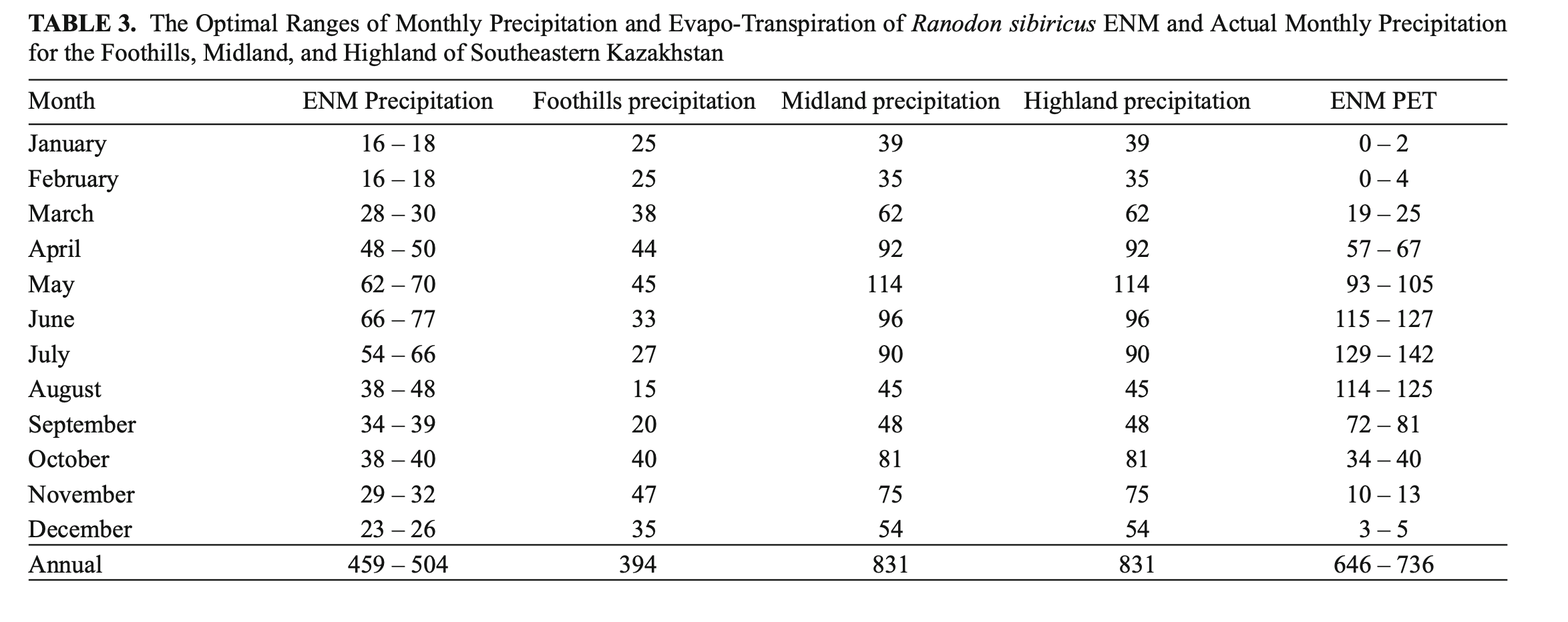
Potential evapo-transpiration. According to the ENM, the annual PET and PET of the months when the species is active exceeded the precipitation mode for the same periods (Table 3). At first sight this pointed to dry land conditions where annual potential evaporation exceeds annual precipitation (Peel et al., 2007). However, sufficient moisture for R. sibiricus habitats is likely to be maintained by a well-developed and highly branched hydrographic network of glacierized basins and the close proximity of underground waters from numerous mountain springs.
Among the key parameters were the PET for January, February, September, and April (Fig. 4). The PET of January and February was minimal (0 – 4 mm) and, with monthly solid precipitation of 16 – 18 mm and mean monthly temperatures of –14°C (Table 3), pointed to stable snow cover preventing deep soil freezing. Snow accumulation in the midland of the Dzungarian Alatau is greatest on the northern slopes. Stable snow cover of up to half of meter lasts from November to April (Seversky I. and Seversky E., 1990) and creates favorable conditions for amphibian hibernation even at very low winter temperatures (Table 2).
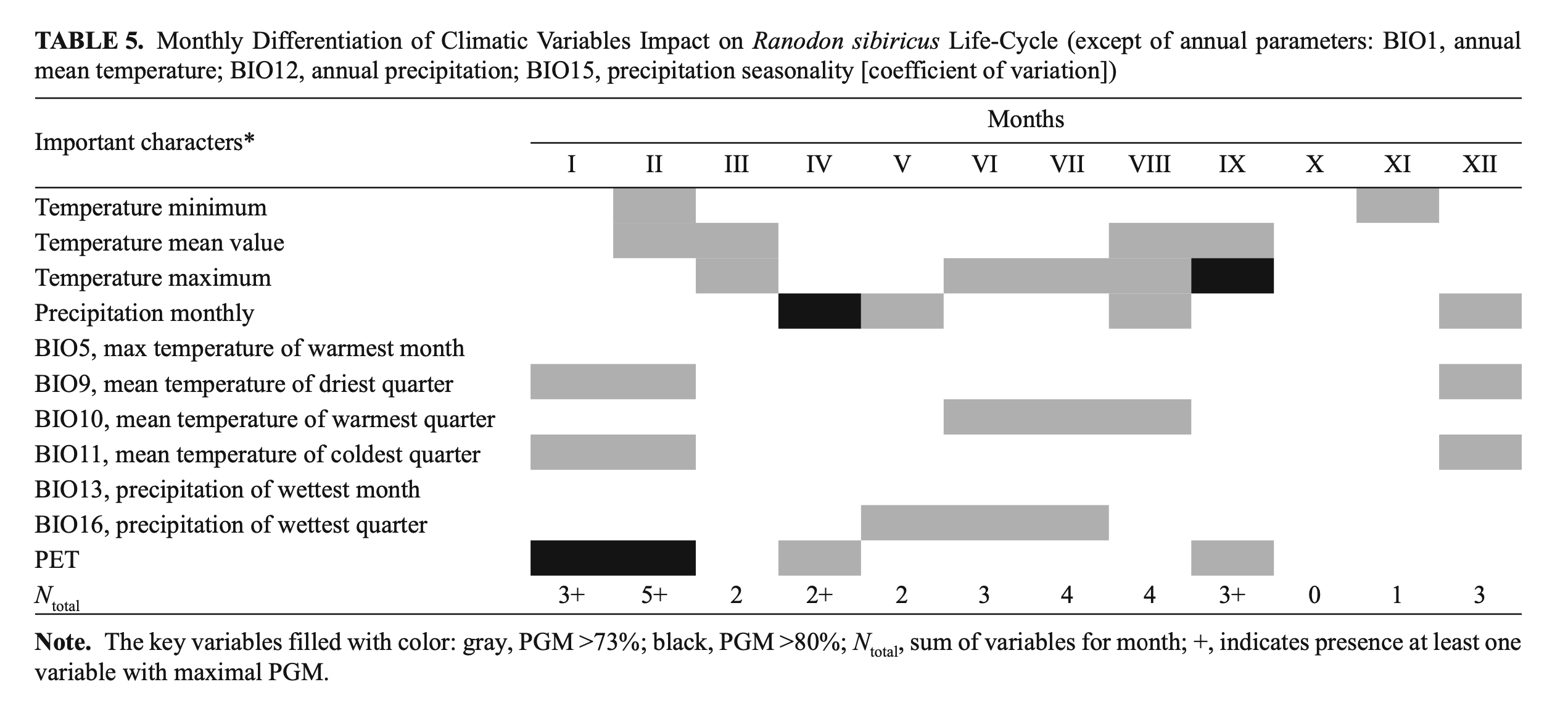
We have undertaken a simple attempt to summarize the contribution of different climatic variables to the R. sibiricus EN. We considered the relevance of every variable in different months of the year and through that tried first to evaluate the importance of every month for the species’ well-being (Table 5). As seen from Table 5, the most important months were from June to September and the winter months (December – February) that coincided, respectively, with the periods of highest activity and deep hibernation of the species.
Our analysis indirectly confirmed a previous suggestion on the importance of glacier mass stability and the steadiness of the annual runoff of mountain watercourses for R. sibiricus‘s well-being (Brushko and Narbayeva, 1988; Dujsebayeva, 2013). Annual precipitation and mean summer temperatures were key parameters of the R. sibiricus EN as a result of their role in maintaining the glacier mass balance (Fujita et al., 2011; Sakai et al., 2015). From the BioClim and WorldClim datasets, we took precipitation in the warm months (May – August) as a key parameter. Seversky et al. (2006) showed the importance of summer precipitation for glacier mass balance in the North Tien Shan and Dzungarian Alatau. Often, summer precipitation freezes and contributes to glacier “conservation,” significantly decreasing ablation. December precipitation is very important for the accumulation of seasonal snow, which is released in the following summer’s runoff. Thus, the position of the Dzungarian Alatau on the border of summer-accumulation and winter-accumulation types of glaciers (Sakai et al., 2015) reinforces to a certain degree our data on the significant role of both summer and winter precipitation in R. sibircus habitat suitability.
Survey effort
In 2015, we inspected several sites in the Kazakh part of the R. sibiricus range to verify the ENM: (I) the western spurs of the Dzungarian Alatau with the Zhangiz and Kusak rivers, (II) the upper and middle flows of the Sredny Usek River, and (III) a small intermontane depression near northern border of the Ili Depression (Fig. 2B).
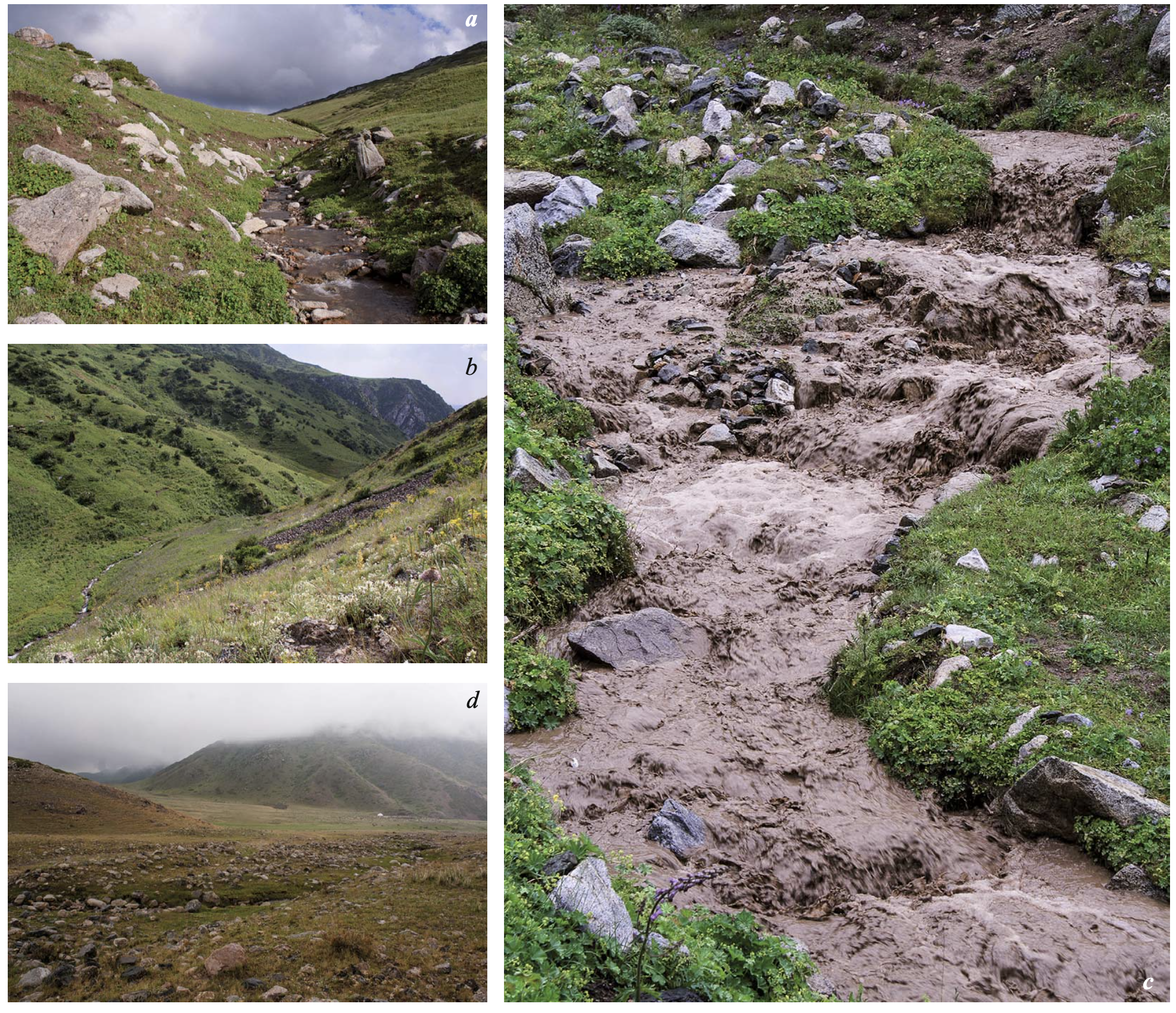
A small mountain, Suyuktobe, was quite suitable for the salamander according to climatic conditions and the precipitation mode, especially (Figs. 6 and 7). However, the model indicated that it might be less suitable orographically (Fig. 3). Our inspection at 1790 – 2600 m a.s.l. revealed that the Zhangiz and Kusak rivers flow in canyon-like narrow valleys and have sinuous rapid streams along most of their length (Fig. 11a, b ). Their highly erosive slopes and significant summer precipitation cause frequent flash floods and mudslides, sometimes completely destroying the watercourses (Fig. 11c). Their close proximity to the deserts of the Balkhash Depression is expressed in warmer summers, stronger evapo-transpiration and hence higher aridity of the landscapes compared with the regions of Dzungarian Alatau to the east. We did not find the salamanders there.
According to the ENM, the Sredny Usek River had neither orographic nor climatic conditions suitable for R. sibiricus habitats (Figs. 3, 6, and 7). We carefully examined its basin in the upper and middle flows (1600 – 2900 m a.s.l.) but did not find the amphibian (Fig. 9b ). A special search for R. sibiricus in the Maly, Sredny and Bolshoy Usek rivers in the 1990s had also been unsuccessful (Kuzmin et al., 1998).
A small intermontane depression in the north of the arid Ili Depression looked quite suitable from the orographic model (Fig. 3) but did not have appropriate climatic conditions (Figs. 6 and 7). It was characterized by sparse water streams, numerous dried-up brooks, and arid landscapes in general (Fig. 11d ). There the salamanders were also absent.
On perspectives of Ranodon sibiricus records in China
The R. sibiricus records known for the upper reaches of the Borotala River Basin (Wang et al., 1992; Kuzmin et al., 1998: Fig. 4; Kuzmin and Tiesmeier, 2001; Chen et al., 2012: Fig. 1) fitted the boundaries of the EN model, apart from Zemaike in the Demekpe River Valley (JMK in Chen et al., 2012). Based on this finding, we have tried a careful appraisal of the probability of new salamander findings in Chinese territory.
Such findings might be possible in the upper and middle reaches of the Urtaksary River (Fig. 7a), but would be less probable in the upper part of Tzigan-Fargi River Valley and in the rivers and brooks flowing into Sairam-Nur Lake from the west. There are areas of suitable orography and forestry there, but the habitats appear quite dry. The Sairam-Nur Depression itself is covered with steppe vegetation and there are few other watercourses in its basin.
The upper reaches of Zolsai Brook and the Kusimchek and Dzherlagan rivers (above 2000 m for the last of these, since below this it flows through a narrow canyon) and the sources of Ashaly River and its left tributaries, as well as the upper flow of Borbosun River, might be checked. Most of these potential habitats are situated quite far from R. sibiricus‘s known range. However, based on the distribution of well-studied endemic species of Carabidae (Coleoptera), these localities are inhabited by representatives of Dzungarian faunistic complex (Kabak, 2014). The morphology of the river valleys and the moisture mode of most of the territory give a some reason to suggest that the salamander could be present here.
Conservation
The ENM gave us reason to evaluate the early ideas on R. sibiricus conservation in situ. In recent decades attempts at species introduction have been undertaken in both Kazakhstan and China. In the 1960s-1970s, the salamander was introduced to some watercourses of the Zailiisky Alatau Ridge (Northern Tien Shan, Kazakhstan), namely Malaya Almatinka River and some brooks in the vicinity of Bolshoy Almatinskoye Lake (1900 – 2000 m a.s.l.). Monitoring in the 1990s pointed to an absence of acclimatized amphibians. The idea of introducing R. sibiricus into the rivers of the Northern Central Ridge (Aksu, Sarkand, Tentek, etc.) or Usek-Khorgos region (Brushko, 1993; R. Kubykin and S. Kuzmin, personal communication) was never realized. In contrast, similar efforts at species introduction were successful in China. The salamanders were transferred from the Sulbeijin River to the Sheunchan locality in the Borotala River Basin (Wenquan Country, Xinjing) and safely settled (Kuzmin et al., 1998 from X. Wang’s personal communication).
Discussing the possibility of R. sibiricus conservation in situ, Kuzmin et al. (1998) wrote about species re-introduction. This seems correct only for Chinese territory. Species resettlement was undertaken within the upper flow of Borotala River Valley, which had suitable relief and partially appropriate climatic conditions according to the ENM (Figs. 3 and 5). The salamander evades the extra aridity of that territory (Fig. 6) by moving higher up in Chinese part of the Dzungarian Alatau compared to Kazakhstan. Historical data show that R. sibiricus populations were more wildly distributed and numerous in the Borotala River Basin not very long ago (Wang et al., 1992; Yuan and Zhang, 2006).
The situation is different in Kazakhstan. A reliable single record of R. sibiricus (or even its ancestor) in the Northern Tien Shan is known only from the Late Pliocene (Averianov and Tjutkova, 1995). A single doubtful record of R. sibiricus in Malaya Almatinka River from the last century (Severtsov, 1873) has not yet been confirmed (Kuzmin et al., 1998). In general, the salamander is not found in the sublongitudinally oriented watercourses of the Northern Central Ridge and Usek-Khorgos region. The ENM confirmed our early suggestion (Dujsebayeva, 2013) that the Northern Central Ridge, Usek-Khorgos region, and the Northern Tien Shan in Kazakhstan were not completely suitable for species introduction, either orographically or climatically (Figs. 3, 6, 7a, b ). Even if the salamander (or more correctly, its ancestor) once lived in these areas, it certainly disappeared during Pleistocene events of orogenesis and glaciation.
We believe that the only correct way for successful in situ salamander conservation in Kazakhstan would be to introduce it to sublatitudinal rivers. This would have to be preceded by analysis of both the current local climate and prediction of its trends under global warming and glacier degradation.
Only regional ecosystems with stable climatic conditions are useful for species conservation in situ (de Castro et al., 2007; Baker et al., 2010). Chen and Chen (2013: Fig. 2) characterized the mountain regions of Central Asia in general and the Dzungarian Alatau in particular as climatically unstable regions. In our previous paper (Dujsebayeva, 2013), we regarded glacier degradation under global warming as one of the main risks for the stability of R. sibiricus populations in near decades. First attempt to predict the changes of the species range point to its possible reduction and continued fragmentation (Dujsebayeva et al., 2014). In the framework of that prognosis, the action on R. sibiricus conservation in situ must necessarily be accompanied by the development of ex situ action. There are currently several examples of successful keeping and breeding of tailed amphibians, including the mountain species, in captivity (Raffaëlli, 2013).
CONCLUSION
The ENM, developed based on GIS analysis, confirmed an uneven spatial and numerical distribution of R. sibiricus in the Dzungarian Alatau Mountains that was determined by oroclimatic and orohydrographic conditions.
Orographically, the ENM accorded with flat or flattish surfaces located on gentle slopes in the midland at 1950 – 2400 m a.s.l. In the Dzungarian Alatau, territories with such characteristics corresponded to intermontane depressions with rivers of sublatitudinal orientation — the Chizhe, Koksu, Borotala, Keskenterek, and Borokhudzir. These rivers possessed the majority of the R. sibiricus populations. Less suitable territories were located in the diagonal valleys of the Kora and Koktal rivers, where the salamander was sporadic and fewer in number. The amphibian was absent from the canyon-like rivers flowing from the Northern and Southern Central ridges as well as from some other regions with watercourses of sublongitudinal orientation. We suspect that such a gross pattern developed under the Alpine orogeny and Pleistocene glaciations but more discussion is beyond the remit of the present paper.
Based on the high percentage of matches between the presence of R. sibiricus and the optimal parameter range, six of the seven orographic variables were referred to as key parameters. Changes in them directly affected salamander habitat suitability andGor appeared to do so through alteration of hydrological and climatic variables. A close interrelation between the relief-forming parameters was revealed.
As regards climate, the ENM belonged within the Humid Continental Climate with short mild-cool summers, relatively cold snowy winters, and all-year precipitation, according to Köppen’s climate classification (DfbGDfc). It was characterized by a July mean temperature of +14.5°C, a January mean temperature of –16.3°C, three months with mean temperatures at or above 10°C, annual precipitation of 459 – 504 mm with higher amounts in summer, and an annual temperature range of 28 – 33°C and corresponded to an aridity index of 30 – 50%. The areas with optimal temperatures were spaced in the midlands of the Dzungarian Alatau quite evenly by longitude and latitude. In contrast, the main territories with optimal precipitation were located in the western part of the Dzungarian Alatau. They decreased in suitability towards the east and were least suitable in the Chinese part of the species range.
For temperature variables we took the following as key parameters: the March mean and maximum temperatures (75% of match), September maximum temperature (85%), the maximum temperature of warmest month (BIO5), and the mean temperatures of the driest (BIO9), warmest (BIO10), and coldest (BIO11) quarters of the year (74%). Among the key parameters for the precipitation variables were April precipitation (81%), annual precipitation (BIO12), precipitation in the wettest month (BIO13), seasonality of precipitation (BIO15), precipitation of the wettest quarter of the year (BIO16) (75%), and May, August, and December precipitation (74%). Temperature and precipitation directly impact the physiological activity of the salamander andGor regulate the onset and manifestation of its phenological phenomena (spring awakening, egg-laying, larval growth and development, hibernation, etc.).
The analysis of relief and climate contribution to EN formation reveals a close interrelation between the two factors. The origin of every watercourse, its hydrological parameters, and microhabitat diversity are determined by local microrelief and microclimate. This gives us cause to talk about oroclimatic and orohygrographic factors. Among the climatic factors, the model accorded greater weight to precipitation than to temperature. Since the precipitation mode in mountain regions strongly depends upon relief we consider the last as a very important abiotic factor defining the spatial and numerical distribution of the species.
The analysis of climatic variables of the ENM confirmed the previous assumption on glacier mass stability and steadiness of the annual runoff of mountain watercourses as important conditions for R. sibiricus‘s wellbeing. Among the key variables of the EN were mean summer temperatures (BIO11), annual precipitation (BIO12), precipitation of the wettest quarter of the year (BIO16), and August precipitation — required to maintain the glacier mass balance.
The results of the EN modeling gave us a reason to discuss critically early ideas on R. sibiricus in situ conservation. According to the ENM, the current orographic and climatic conditions of sublongitudinal rivers flowing from the Northern Central Ridge, Usek-Khorgos, region and Northern Tien Shan are not suitable for the species in many aspects. It seems more realistic to search the sites in sublatitudinal river valleys. Concomitant analysis of current climatic conditions and their future changes under global warming and glacier degradation is necessary. The present instability of the climate in the Dzungarian Alatau determines the reality of species ex situ conservation as well.
We consider the ENM that we created as a primary and basic pattern because of limits set on the analysis. We considered only abiotic factors such as relief and climate and not all of the variables important for R. sibiricus‘s life cycle, for example, the presence of permanent and temporary water streams inside the suitable area or water chemistry. We also did not touch on biotic factors like the abundance of food or relations with competitor and enemy species. This enumeration can be continued further in accordance with the understanding of the ecological niche as an n-dimensional abstract space, each axis of which corresponds to a certain parameter (Hutchinson, 1957).
Acknowledgments. The research was partially supported by grants from the Ministry of Education and Sciences of Kazakhstan (2009 – 2011), Stiftung Artenschutz’s “Amphibian Funds” in cooperation with the Verband Deutscher Zoodirektorene (VDZ) and co-funding zoological gardens (2012), and the GEF Small Grant Program (2014). “STeK” Limited Liability Company (Tekeli), the Institute of Geography (Almaty), and the Association for the Conservation of Biodiversity of Kazakhstan (Astana-Almaty) provided financial and technical support in different stages of the research.
The authors thank deeply the colleagues and other volunteers who participated in our expeditions in the Dzungarian Alatau in different years: Andrey Kovalenko, Oleg Okshin, Oleg Belyalov, Dmitry Sagurov, Ulan Dosbolov, Sergey Gaidin, Gennedy Dyakin, Irina Arifulova, Juliya Zima, Vladimir Kazankov, Rustam Khakimov, Natalia Naumenko, Rakhim Shukurbayev, Tatjana Piterova, Sergey Kalesnikov, Nikolay Postnikov, Fedor Gaivoronsky, Evgeny Eshel, Erkebulan Khalikov, Adilet Valeyev, Gabit Esimbekov, Saule Uksukbayeva, Arauzhan Bekkulieva, and Dmitry Cheremnov. Dmitry Sagurov organized two walking trips to verify the ENM in hard-to-reach regions of the Dzungarian Alatau. The hospitable house of Mariya and Dmitry Sagurov in Tekeli town served as the base camp for our expeditions throughout the research years. We thank Daniil Berman for the idea of conducting a field experiment to determine the temperature conditions in potential sites of R. sibiricus hibernation (manuscript in preparation); Aldar Gorbunov for discussion of the problem of soil freezing in the mountains; Vladimir Aizen, Evgeny Vilesov, and Alexander Kokarev for consultations on mountain glacier dynamics; Alexander Trifonov and Azhar Eszhanova for advice and correction of our geomorphological interpretations; and Oleg Podrezov and Alexander Cherednichenko for discussion on mountain climatology problems. Ilya Kabak shared long-term landscape observations in Xinjiang and ideas on zoogeographical zoning of the Dzungarian Alatau and Tien Shan. We are grateful for the essential critical remarks made by Daniil Berman, Vladimir Ischchenko, and Evgeny Dunayev during the preparation of the manuscript and Cathy Lowne for her kind help with English correction.
REFERENCES